Citation: Vilaplana M, Vasiev A, White S, “Function-Based Primary Packaging Design for Injecting Viscous Non-Newtonian Formulations”. ONdrugDelivery Magazine, Issue 101 (Oct 2019), pp 20-25.
Marta Vilaplana; Alex Vasiev and Susie White explore the challenges of delivering high-viscosity, non-Newtonian fluids emphasising the importance of robust tools to characterise specific device and formulation parameters in light of increasing regulatory scrutiny.
“As the biologics and LAIs markets grow, there is greater scrutiny of the interaction between biologic/drug products and primary drug containers.”
Recent years have seen a rise in high-viscosity formulations within parenteral drug delivery. This change can be attributed to the increasing development of biologics – which offer greater specificity but tend to have high molecular weights – and the increasing popularity of long-acting injectables (LAIs).
Formulating LAIs involves creating a physical barrier around the active pharmaceutical ingredient which slows its release. This can be achieved by dissolving it in highly viscous, slow-absorbing oil or containing it within hydrogels or microcarriers – methods which often result in a product with unusual or challenging flow characteristics. The release of therapeutics at a controlled rate over a period of weeks or months benefits both patients and healthcare providers. LAIs reduce dosing frequency, which improves convenience for patients and reduces healthcare costs.
LAIs exhibit some or all the following characteristics that make them very difficult to deliver:
- High viscosity
- Drug in suspension
- Non-Newtonian behaviour (e.g. shear thinning)
- Propensity to clog due to large particulate sizes
- Propensity to settle during storage
- High sensitivity to environmental conditions
- Need for depot formation to control pharmacokinetics
Consequently, there are several factors that designers need to consider when designing an autoinjector and selecting a primary drug container for these formulations.
STANDARD PRIMARY PACKAGING AND AUTOINJECTOR DESIGN APPROACH
As the biologics and LAIs markets grow, there is greater scrutiny of the interaction between biologic/drug products and primary drug containers, including stability during shelf life and particulate burden, among other critical quality attributes.
The design of an autoinjector is also largely determined by the selection of the primary drug container – a decision which occurs early in the drug development process. Traditional primary packaging formats may not be ideal for the application and may put the future device at a relative disadvantage.
Considerations for Glass Containers
In previous years, recalls have increased due to quality issues in primary glass containers leading to breakage or delamination. As more attention is paid to the integration of primary drug containers with autoinjectors to form combination products, finding solutions to these problems has taken on an increased urgency – to protect patients and ensure the integrity and proper delivery of medication.
Degree of Concern Associated with the Route of Administration | Likelihood of Packaging Component-Dosage Form Interaction | ||
High | Medium | Low | |
Highest | Inhalation Aerosols and Solutions; Injections and Injectable Suspensions+ | Sterile Powders and Powders for Injection; Inhalation Powders | |
High | Ophthalmic Solutions and Suspensions; Transdermal Ointments and Patches; Nasal Aerosols and Sprays | ||
Low | Topical Solutions and Suspensions; Topical and Lingual Aerosols; Oral Solutions and Suspensions | Topical Powders; Oral Powders | Oral Tablets and Oral (Hard and Soft Gelatine) Capsules |
+ For the Purpose of this table, the term suspension is used to mean a mixture of two immiscible phases (e.g. solid in liquid or liquid in liquid). As such it encompasses a wide variety of dosage forms such as creams, ointments, gels and emulsions, as well as suspensions in the pharmaceutical sense.
Table 1: Illustration of degree of concern regarding the route of administration with the likelihood of packaging component-dosage form interactions.2
The US FDA rates the level of concern of a given primary pack, based on route of administration and drug type (Table 1). Packaging for injection is ranked as the highest concern category based on risks associated with route of administration and packaging-drug reactivity.1 This gives more emphasis to the importance of the design considerations relating to primary drug containers. Additionally, its “Immunogenicity Assessment for Therapeutic Protein Products” called attention to issues commonly associated with container closure systems, including:
- Denaturation and aggregation of proteins at glass and air interfaces
- Delamination and particulate formation in certain formulations
- Protein aggregation associated with silicone-lubricated containers
- Leachables from container components.
“Traditional filling methods limit the needle and mechanism options available to the designer.”
Glass primary packaging is an ideal choice when the focus is a well-known regulatory pathway, well-defined stability characteristics and low cost. Two common glass primary drug containers are glass cartridges (e.g. lidocaine and insulin) and staked-needle prefilled syringes (e.g. heparin and adalimumab). However, glass is brittle, with its failure defined by the weakest link or most critical defect present on the surface.
Critical defects arising through abrasion during manufacture reduce the pressure resistance of a glass primary container and ultimately limit the pressures that a high-viscosity autoinjector can generate. This is especially true if a pressure spike occurs as the autoinjector impacts the container at the start of delivery. Specialised processes and coatings for glass containers are now offered by some producers, who claim a threefold improvement in breakage resistance.2 However, these processes inevitably offset some of the low-cost advantage attributed to glass.
Traditional filling methods limit the needle and mechanism options available to the designer. Filling a highly viscous fluid into staked-needle syringes can introduce voids, which then foam and expand when a vacuum is applied during stoppering. The bubble introduced during back filling also poses an issue for biologics, which tend to agglomerate at the gas-liquid interface. Tip filling, common to dental cartridges, virtually eliminates voids and bubbles but is limited by the accuracy of the filling equipment and the design of the primary drug container.
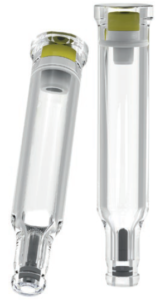
Figure 1: Oval’s COC primary drug container technology optimised for high-pressure, high-viscosity delivery.
Glass Interaction with the Autoinjector
Both glass cartridges and syringes have limited features for accurate location within an autoinjector. Glass syringes are generally held by their shoulder or a flange formed at the back of the container, the shapes of which are not well defined due to the limitations of the glass-forming process. In an autoinjector, this leads to tolerancing issues and high variability of inserted needle depth. These can also introduce stress concentrations that weaken the component. Glass cartridges typically rely on their neck for axial position. This applies load to a point where residual stresses from manufacturing operations can increase the risk of failure.
A NEW APPROACH TO DRUG PRIMARY PACKAGING AND AUTOINJECTOR DESIGN
“Developing robust tools and methods to characterise specific parameters that are the focus of regulatory concern is key.”
An Alternative to Glass
Oval Medical Technologies has developed a cyclic-olefin copolymer (COC) primary drug container for high-viscosity drugs (Figure 1), which resolves many of the aforementioned issues with glass primary packaging and autoinjector design. This primary drug container can tolerate significantly higher pressure and, unlike glass, it does not experience catastrophic failure. It also incorporates robust positioning and bubble-reducing features, offering flexibility for tip or back fill. Table 2 compares Oval’s high-pressure COC primary container with typical glass primary packaging commonly used in autoinjectors.
Oval High Pressure COC Primary Drug Container | Glass cartridge | Prefilled glass syringe | |
Locating features for handling and positioning in device | Custom locating features and tight tolerances | Shoulder or neck of the vial, crimp | Flange of the syringe |
Fill options | Customisable Tip or Back fill (with low bubble volume) | Tip or Back fill | Back fill |
Lubrication | None required | Baked on silicone and stopper lubrication (various) | Spray-on silicone lubrication and stopper lubrication (various) |
Needle | Dry needle | Dry needle | Staked needle |
Oxygen permeation resistance5 |
Poor (Excellent with co-injected barrier layer) |
Excellent | Excellent |
Vapour permeation resistance |
Good6 (Excellent with co-injected barrier layer) |
Excellent5 | Excellent5 |
Maximum operational pressure limit | 100-150 bar | ~20-60 bar7 | <75 bar8 |
Impact resistance5 | Excellent | Poor5 | Poor5 |
Failure mode | Plastic deformation | Brittle fracture | Brittle fracture |
Table 2: A comparison of Oval’s high-pressure COC primary drug container with typical glass primary packaging commonly used in autoinjectors.
Several technologies are now available to modify the drug contact material where COC is not appropriate, including vapour deposition of glass-like coatings.3,4
Function-Based Approach to Primary Drug Container Design
Oval’s primary drug container designs are the result of years of development, and a thorough understanding of all the functions, considerations and requirements that the primary drug container must incorporate to safely store and deliver drug products with potentially challenging characteristics.
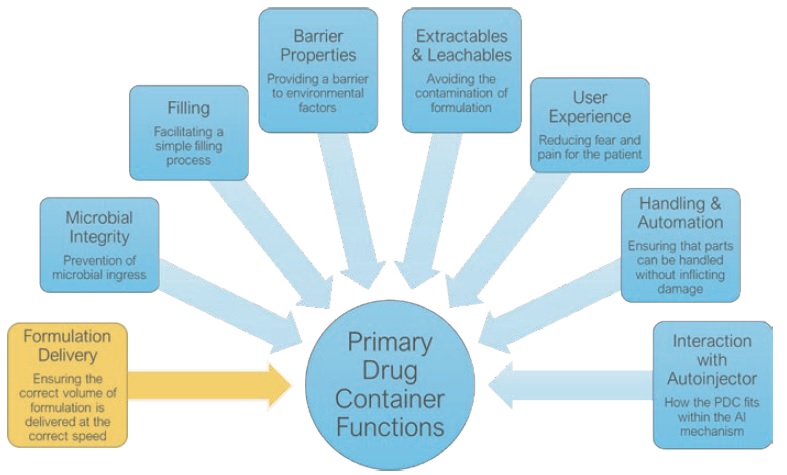
Figure 2: An illustration of key attributes that shape and define the specifications of each primary drug container function.
We characterise the requirements shown in Figure 2 in the context of the drug product properties and user needs. This process involves multiple tools and resources, some of which are well known within the industry (mathematical modelling), whilst others have been fully developed by Oval (Injection Characterisation System). Using these tools to investigate variables in the context of specific primary drug container functions allows them to be translated into tangible design features, such as the geometry and materials.
This article provides context to the choice of tools used at Oval to characterise the myriad variables that play a role in understanding formulation delivery (Table 3).
Primary Drug Container Function | Tools & Resources | Variables | Outputs |
Formulation Delivery | • Mathematical models • Monte Carlo simulations • Proprietary Injection Characterisation System (ICS) • Rheology |
• Delivery force • Delivery speed • Needle geometry • Shear rate • Temperature • Volume • Formulation viscosity • Formulation |
• Spring rate / geometry • Optimum delivery force • Safe system pressure • Primary Drug Container material selection • Optimum needle geometry • Predicted/real delivery time & rate • Formulation |
Table 3: Oval’s approach to understanding formulation delivery involves the use of various tools to characterise variables and identify outputs that help define primary drug container design.
UNDERSTANDING FORMULATION DELIVERY
Understanding the physical characteristics of a drug formulation early in the product development process is of the utmost importance. It increases the likelihood of achieving the required specification and reduces the risk of unforeseen technical challenges later in development.
Formulation viscosity is often determined with a viscometer or rheometer – but these measurements have limitations. Care must be taken to ensure that the shear rate at which testing is performed is representative of that seen during injection. For spring-powered high-viscosity autoinjectors, this varies during the stroke and is likely to be between 2,000 and 100,000 s-1. Whilst certain rheometers can achieve these shear rates, they are outside the scope of much viscosity testing. Exposing these fluids to the environment can also lead to a loss of solvent and impact the measured value.
Formulations can behave differently within an autoinjector than might be predicted from rheometer data alone. Unforeseen difficulties in delivery may only become apparent when injecting with a needle and syringe (e.g. settling of a suspension).
Large particle sizes and aggregation can cause serious problems such as clogged needles or irregular flow. Separation of suspensions from a carrier fluid can cause non-homogenous delivery, variability in delivered dose and altered pharmacokinetic profile.
Describing Non-Newtonian Fluids
Many high-viscosity formulations exhibit shear-dependant behaviour defined by various models including power law, Carreau and others. Power law fluids, which are the focus of this article, demonstrate a time-independent log-log relationship between shear stress and viscosity described by:
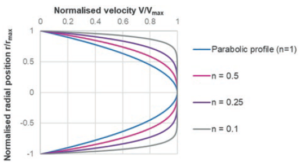
Figure 3: Illustration of a power law fluid velocity profile in a needle depending on the flow behaviour index n.
Where du/dr is the flow velocity gradient, μ0 is the flow consistency index which describes the low-shear viscosity and n is the flow behaviour index. Unlike Newtonian fluids which form a parabolic flow profile in a needle (Figure 3), the flow profile of power law fluids is defined by the expression for flow velocity u at a given radial location r:
Where R is the maximum internal radius and dp/dl is the pressure gradient along the length of the needle. This creates a unique volumetric flow rate Q at a given pressure (applied force F and container internal bore D):
Where d is needle internal diameter, and l is the needle length.
Injection Characterisation System
Oval’s proprietary Injection Characterisation System (ICS) overcomes issues associated with traditional rheological formulation characterisation by performing it in situ. The set-up of the ICS is like that of an autoinjector, with the addition of a range of instrumentation to provide feedback on the injection process.
It consists of a syringe attached to a needle via a pressure transducer to monitor internal pressure within the syringe. Behind the syringe is a plunger rod connected to a linear encoder, tracking the rate of delivery. This is powered by a spring which acts on a load cell to monitor the force needed for delivery. Combining the outputs of these sensors provides a huge range of information about the formulation.
Apparent formulation viscosity is determined for a wide range of tests (varying needle gauge and length, spring force, etc.) and environmental conditions. Changes in the formulation arising from recipe changes, processing and ageing can also be detected, giving an indication of the impact of batch-to-batch variation or comparing different candidate formulations for injectability.
Closely approximating the delivery system at an early development stage helps detect and mitigate unforeseen risks.
Using Drug Characterisation to Define Mathematical Models
The force of springs traditionally used in autoinjectors varies depending on the level of spring compression. The spring force at a certain spring length F is defined by:
Where F0 is the initial force, k is the spring rate constant and x is the displacement of the spring in axial expansion. Substituting the spring force relation into the previously defined flow rate equation and integrating over the delivery stroke provides a relation for injection time:
Where L is the delivery stroke (mm).
This allows injection time for a power law fluid to be calculated from the starting force and the delivery stroke (Figure 4).
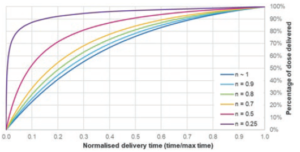
Figure 4: Illustration of power law constants’ effect on delivery profile. Assumptions: μ0 = 1000 mPa sn. D = 8.65 mm, l = 20 mm, d = 0.34 mm F0 = 45 N, k = 1.5 N/mm, Fmin = 5 N.
Application to Monte Carlo Simulations
With injection time represented by a simple mathematical relation, a Monte Carlo simulation of delivery times can be performed (Figure 5). Each input variable is described by an appropriate statistical distribution generated from supplier specifications, predicted process capability or characterisation of device components. Through random sampling from the input variables, the simulation generates predicted delivery time for millions of simulated devices.
This process allows the impact of needle and spring combinations to be evaluated early in the development process. Verification of the model is done using physical testing of the formulation in the appropriate context (lab-based injection rigs or prototype devices). Power law formulations characterised in the ICS show a high degree of correlation between real and simulated deliveries. Due to confidentiality and the unique behaviour of the formulations, these results cannot be shown.
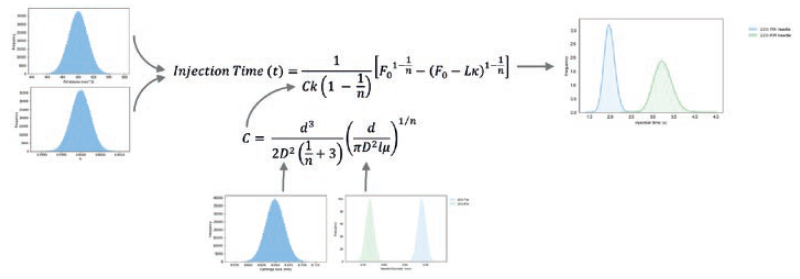
Figure 5: Process of needle optimisation through Monte Carlo simulation of a power law fluid.
A ROUTE FORWARD
The increase in regulatory scrutiny on primary packaging requires a more proactive role on the part of pharmaceutical/biologic companies to address the various concerns and considerations associated with standard approaches. Developing robust tools and methods to characterise specific parameters that are the focus of regulatory concern is key. Establishing partnerships with primary packaging manufacturers early in product development may help create unique solutions to the challenges of LAIs, biologics and injectable drug packaging that are otherwise detected too late in the process.
REFERENCES
- “Guidance for Industry,Container Closure Systems for Packaging, Human Drugs and Biologics”. US FDA, May 1999.
- “Fina Cartridges”. Company Web Page, Stevanato Group, Sep 2019. (https://pharma.stevanatogroup.com/glass-primary-packaging/products-platforms/cartridges/#fina-cartridges)
- “Technology Overview”. Company Web Page, SiO2 Medical Products, Sep 2019 (http://www.sio2med.com/technology.html)
- “Coating of COC”. Company Web Page, Micronit, Sep 2019. (https://www.micronit.com/microfluidics/Coating-of-COC.html)
- Forcinio H, “Selecting Primary Packaging for Parenterals”. Pharm Tech, 2018, Vol 42(7), pp 46–50.
- “SCHOTT TopPac® – Polymer Prefillable Syringes”. Company Web Page, Schott, Sep 2019. (https://www.us.schott.com/pharmaceutical_packaging/english/products/syringes/polymer_syringes.html)
- “Burst Pressure & Fracture Mechanics of Technical Glass Tubing”. Company Web Page, Schott, Sep 2019. (https://www.us.schott.com/tubing/english/products/properties/burst-pressure.html)
- Wohland A, et al, “Encoding and Reading of Codes on Glass Containers for Pharmaceutical and Diagnostic Products” Die Pharmazeutische Industrie, 2009, Vol 7(10), pp 1770-1774.