To Issue 156
Citation: van Ginneken T, “Delivery Solutions for Deep-Cold Drugs.” ONdrugDelivery, Issue 156 (Jan 2024), pp 78–82.
Tom Van Ginneken considers how the growing pipeline of molecules that require cold storage has highlighted the need for better drug containment and delivery methods and highlights the potential SCHOTT TOPPAC® freeze syringes offer as a potential solution.
In recent years, there has been strong growth in drugs that need to be stored at low temperatures to remain stable. Messenger RNA (mRNA) vaccines are a notable example, developed during the covid-19 pandemic, and with potential to be used for other therapeutic applications, including influenza, respiratory syncytial virus and other infectious diseases. The US FDA has approved eight therapies across three different types of viral vectors: adeno associated virus, lentivirus and herpes simplex virus. To date, more than 20 cell and gene therapies have been approved, 25 viral-vector therapeutics are in late-stage development, and 120 have reached Phase II trials.
“The primary concern is always patient safety, and there are three key areas where deep-cold storage could pose challenges – syringe functionality, CCI and drug stability.”
This represents an expanding pipeline of sensitive molecules that need to be stored at temperatures from -20°C down to -196°C to maintain drug stability. It is clear that current packaging products are not ideal for this purpose and that today’s deep-cold drugs require a better containment and delivery solution.
Currently, these molecules are packaged either in vials or cryobags, but neither method is entirely satisfactory. In the case of vials, the drug transfer to a syringe requires a number of manual preparation steps. This is not only time-consuming but also increases the risk to patient safety through contamination or dosage errors. Cryobags are difficult to fill and are more susceptible to breakage or issues with container closure integrity (CCI).
Next-generation products are needed that can offer a combined storage and injection system, reduce the risk of breakage and CCI issues, and enable an easier and faster drug delivery process with less opportunity for medical errors. Prefilled syringes (PFSs) meet all these criteria, but their use in deep-cold storage also raises a number of questions. At extremely low temperatures (-100°C), rubber may lose elasticity, the materials used for a PFS may experience shrinkage at different rates, amounts of leachable silicone may increase (creating additional particles) and lipid nanoparticle (LNP) stability may be affected.
The primary concern is always patient safety, and there are three key areas where deep-cold storage could pose challenges – syringe functionality, CCI and drug stability. Before it reaches the patient, an mRNA vaccine in a PFS is frozen and will often have undergone one or more air transport cycles.
During preparation, storage and transportation, there may be multiple occasions where environmental forces can affect the device, and particularly its plunger, causing it to move within the syringe barrel. For example, when the PFS is frozen, the liquid formulation may expand, causing the plunger to move away from the distal (cone) end of the device or, conversely, move towards the needle should any air trapped in the syringe contract upon freezing. Whereas, during air transport, the atmosphere within an unpressurised aircraft hold, which may be half the pressure at ground level, could mean that the plunger moves away from the needle-end. In combination, the effect of these forces is difficult to predict and, once the plunger movement exceeds the distance between the first and last sealing lip, it is defined as breaching the sterility barrier, which presents a risk to patient safety.
PLUNGER MOVEMENT AT LOW TEMPERATURE
SCHOTT Pharma performed a design of experiments (DoE) with different plunger materials, filling volumes, headspace sizes, filling media and freezing temperatures on SCHOTT TOPPAC® freeze 1 mL cyclic olefin co-polymer (COC) syringes (Figure 1) to evaluate the impact of these variables. The maximal plunger movement was determined for filled syringes that were frozen for 12 hours with one or more freezing cycles and at reduced ambient pressure, simulating the worst case of air transport in an unpressurised aircraft.
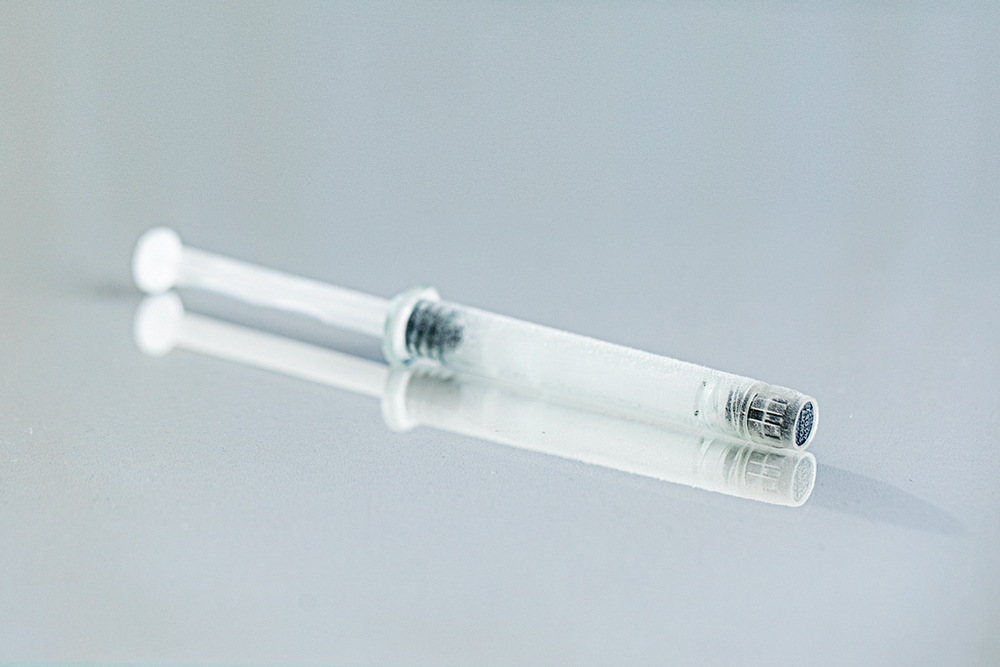
Figure 1: SCHOTT TOPPAC® freeze 1 mL syringe for deep-cold temperatures.
Three factors were shown to have a significant influence on plunger movement (Figure 2). The first of these was the fill volume. The higher the fill volume, the more it expands during freezing and the greater the resulting movement of the plunger. Second, the amount of headspace also affects the degree of plunger movement – during freezing, the air will contract in volume and force the plunger to move towards the cone, while the force of the under-pressure of the air transport simulation will push the plunger towards the flange.
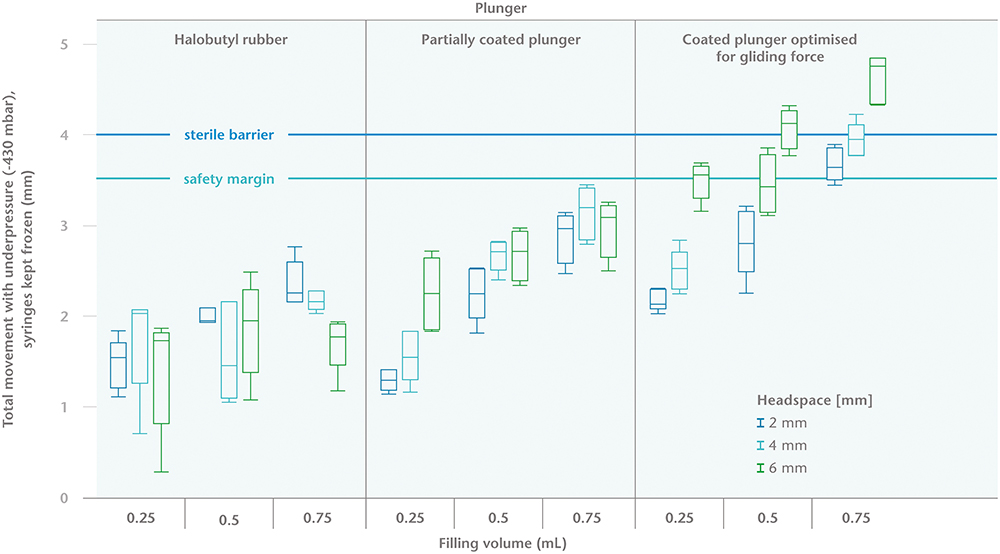
Figure 2: Maximal plunger movement (in mm) during storage at -20°C and at an under-pressure of 430 mBar.
The third factor is the material and the design of the plunger itself. Plungers that are optimised for break-loose and gliding properties will show greater movement. Factors that could influence the gliding properties include geometry, compression set, overlap with barrel, low friction coatings and sterilisation mode. Furthermore, the plunger’s design impacts the sterile barrier, and it should be noted that the distance between the first and last sealing lip is not identical for all plungers. Therefore, it is important to understand each parameter to ensure the optimum selection of plunger material and design to suit the application.
Two other parameters were investigated as part of the DoEs – drug solution, in which water for injection (WFI) was compared with an mRNA placebo of 8.7% sucrose solution; and three different freezing temperatures: -20°C, -50°C and -80°C. Neither parameter was found to have any significant effect on plunger movement.
The main observation of the study is that choosing the correct primary packaging and filling parameters can control plunger movement and avoid breaching sterility during transportation and deep-cold storage. The recommendations are that a plunger with a higher break-loose and gliding force should be used, headspace should be reduced as far as possible and should not exceed 4 mm, and the fill volume should preferably be ≤0.5mL.
EFFECT OF FREEZE/THAW
It is also important that the syringe performance is not impaired by low temperature storage conditions. The syringe system would be useless if freezing increases the likelihood of breakage or hinders the plunger movement after the freeze/thaw cycle. SCHOTT Pharma therefore performed studies to compare polymer syringe functionality after freezing with syringes stored at room temperature to ascertain the influence of temperature, headspace and plunger type on break-loose and gliding forces.
Syringes were filled with WFI and subjected to three cycles of freezing to deep-cold temperatures and thawing to room temperature. The break-loose and gliding forces were tested at room temperature. No difference in break-loose and gliding forces was observed between -20°C, -50°C and -80°C storage, indicating that the temperature of the freeze/thaw process does not significantly affect the forces needed to perform the injection. Headspace also appeared to have no impact on break-loose and gliding forces.
Due to the stickiness effect of the rubber, break-loose forces tend to increase over time. In this application, because of the plunger movement during freeze/thaw, the thawing process already forces the plunger to move and, therefore, little increase in break-loose force is observed.
However, the plunger type did have a significant effect on the injection forces. Different types of plungers have different gliding profiles and characteristics. As expected, the plunger that was developed with improved gliding properties in mind performed the best.
“While silicone oil is normally not toxic to the human body, an abundance of it can create subvisible particles that could react with the drug and reduce efficacy.”
CCI AT LOW TEMPERATURES
The second important question arising from deep-cold storage conditions is whether CCI is maintained at extremely low temperatures, guaranteeing a barrier against microbial ingress. A PFS consists of multiple components: a closure for the cone side, the plunger and the syringe barrel. These components may be made from a broad range of materials, such as glass or polymer for the syringe barrel and chlorobutyl or bromobutyl for the rubber components. Furthermore, various coatings can be used on the syringe barrel and plunger. All these materials have different thermal expansion coefficients and will shrink at different rates when frozen. An acceptable compromise will be necessary between good sealing properties and gliding forces because a reduced sealing overlap between plunger and barrel will drastically increase the risk of CCI issues.
Under mRNA-specific cold chain conditions, for example, the inner diameter of a COC syringe shrinks by roughly 0.044 mm while the rubber plunger’s outer diameter shrinks by 0.050 mm, 0.047 mm and 0.040 mm for the three respective sealing lips. Since the shrinkage rate of the COC material is closely aligned to that of the rubber components, the risk of loss of overlap between syringe barrel and plunger, and therefore a CCI breach, is minimised, even at temperatures of -80°C (Figure 3). On the other hand, the thermal expansion coefficient for Type I glass is at least a factor of 10 smaller than COC or the rubber components. If glass is used as the primary packaging material, the container will shrink at a much lower rate than the rubber plunger, reducing the sealing overlap and potentially posing a risk of leakage or microbial ingress.
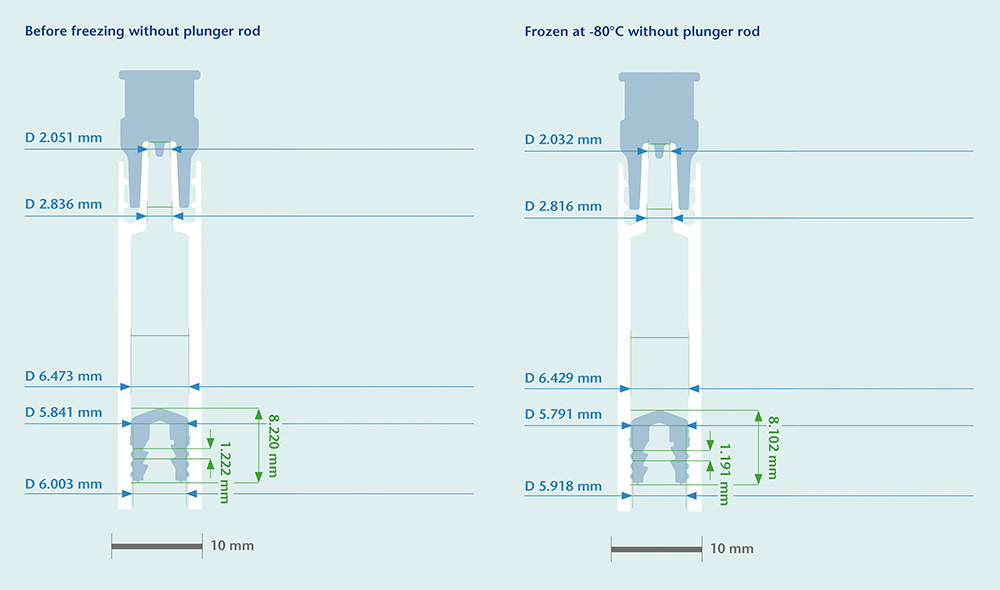
Figure 3: Computed tomography (CT) scan of identical COC syringe systems at different temperatures.
An external headspace study was also carried out to investigate further the reduced sealing overlap. Fifteen empty COC syringes were stored for 24 hours on dry ice at -80°C, with temperature excursions up to -100°C, then tested with a qualified Lighthouse Instruments (VA, US) FMS-carbon dioxide headspace analyser (Model FMSCO2). Measuring the amount of CO2 in the headspace enables any leakage during cold storage to be identified.
Different positive control samples – syringes with laser-drilled holes in the plunger, or a needle or microwire bypassing the sealing lips of the plunger – were used to confirm the test method. The cone closure and the plunger were also individually tested to locate the source of any leakage. None of the 15 samples showed signs of CO2 ingress, indicating that, even at -100°C, the COC syringe system maintains CCI. Furthermore, CCI was maintained even after prolonged storage.
SILICONISATION AND DRUG STABILITY
The third element that may affect patient safety as a result of very low temperature storage is drug stability. A standard PFS has a lubrication layer of sprayed-on silicone oil to allow the plunger to move inside the barrel. While silicone oil is normally not toxic to the human body, an abundance of it can create subvisible particles that could react with the drug and reduce efficacy. Determining the amount of leachable-free silicone gives an indication of the potential risk of drug-lubricant interaction.
SCHOTT Pharma conducted a study comparing standard silicone-oil spraying and a cross-linked silicone technology. For each variation, five SCHOTT TOPPAC® freeze syringes were filled with WFI and subjected to either three freeze-thaw cycles at -20°C or storage at 5°C. The extract from the five samples was pooled and analysed by graphite furnace atomic absorption for free silicone oil. This test method has a detection limit of 0.2 mg/L.
The study showed clear differences between the two lubrication technologies. Even for the reference samples stored at 5°C, the leachable silicone amount for sprayed-on silicone oil was at least five times higher than for the SCHOTT TOPPAC® freeze syringes using cross-linked silicone. The results were even more pronounced at lower temperatures. After three freeze-thaw cycles at -20°C, the leachable silicone quantities increased for both technologies, but 24 times more free silicone oil was observed for the syringes with sprayed-on silicone (Table 1). The results collected at -20°C are likely to apply to temperatures down to -80°C as the thermodynamic phase transition (crystallisation) effect of the freezing process puts stress on the lubrication layer rather than the temperature itself.
Time | Free silicone (mg/L) 3x frozen at -20°C and thawed |
Free silicone compared with standard SCHOTT TOPPAC® | Free silicone (mg/L) stored at 5°C |
Free silicone compared with standard SCHOTT TOPPAC® | |
SCHOTT TOPPAC® cross-linked siliconisation Standard cross-linked silicone |
0d | 0.23 | N/A | <0.2 | N/A |
SCHOTT TOPPAC® spray siliconisation Sprayed on DC360, 0.55 mg/barrel |
0d | 5.6 | 24 TIMES | 1.09 | 5 TIMES |
Table 1: Leachable-free silicone quantities of two siliconisation technologies.
The choice of siliconisation technology also has a significant impact on subvisible particles at freezing temperatures. Lower storage temperatures seem to increase particle load, and this effect seems to increase over time. The immobilised silicone layer from cross-linked siliconisation showed the lowest particle levels at freezing temperatures over storage periods of 12 hours, 31 days and 121 days when compared with sprayed-on silicone oil, although it should be noted that all results were within those stipulated in US Pharmacopeia <788>. There was a high probability that all subvisible particles observed originated from siliconisation – a silicone-free syringe used as a control showed no particles.
It is clearly important to consider the impact of siliconisation technologies on a specific drug application as there could be consequences for drug stability. The results of this study indicate that cross-linked siliconisation technology for deep-cold applications offers superior results in leachable silicone quantities and sub-visible particle burden. This cross-linked siliconisation process is standard for the SCHOTT TOPPAC®COC syringe portfolio, and not only provides good drug stability properties but also stable gliding performance at different storage conditions.
PRIMARY PACKAGING COMPATIBILITY
A further consideration relating to drug stability is the compatibility of the primary packaging with the LNPs that are used to encapsulate the mRNA and protect it while it travels to the dedicated cells to perform its therapeutic function. The stability of these LNPs is vital to maintaining the efficacy of the mRNA. SCHOTT Pharma selected three analytical methods to characterise the syringe compatibility with LNPs: LNP particle size, polydispersity index (PDI) and LNP adsorption.
In tests carried out in collaboration with the Institut für Pharmazeutische und Biomedizinische Wissenschaften (IPBW) at the University of Mainz (Germany), SCHOTT TOPPAC® freeze syringes were compared with glass vials after storage at -80°C and for different ageing time points. LNP particle size was measured using a dynamic light scattering technique from Zetasizer (Malvern Panalytical, UK), and no significant difference was observed between the solution stored in a glass vial or that in a polymer syringe.
The same technique was used to measure the PDI, which gives an overview of the homogeneity of the particle size and provides feedback on particle stability and/or potential agglomeration. The lower the PDI, the lower the particle size distribution, and, therefore, the higher the LNP stability, with values of 0.3 and below most commonly deemed acceptable for drug delivery applications using lipid-based carriers. When SCHOTT TOPPAC® freeze syringes were compared with glass vials after storage at -80°C, once again, no significant difference was observed between LNP PDI in solution stored in a glass vial or a polymer syringe.
LNP adsorption on the inside surface of the container can reduce the concentration of the API, with a lower adsorption level indicating the container’s chemical inertness. Again, tests were carried out for market standard glass vials and SCHOTT TOPPAC® freeze at storage temperatures of -80°C and 2–8°C and for different ageing time points.
The inner surface of the SCHOTT TOPPAC® freeze syringes and glass vials were analysed, and deposits identified using time-of-flight secondary ion mass spectrometry (ToF-SIMS). A multivariate curve resolution technique was applied to extract a pure component spectrum from a ToF-SIMS spectrum to enable the selection of important fragment ions of each material in a sample. These pure spectra could then be matched with signal patterns expected for the analysed sample (e.g. silicone). A lower LNP adsorption effect was noticed for polymer syringes compared with glass vials (Figure 4).
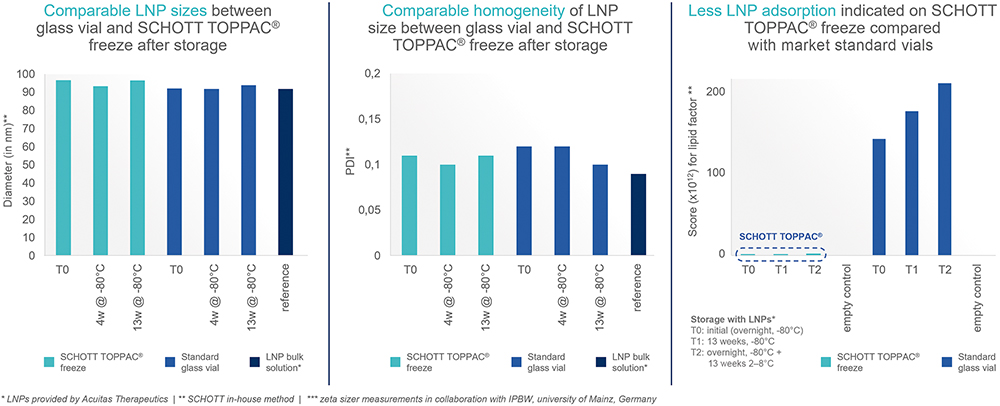
Figure 4: Compatibility data for LNPs at least as good for SCHOTT TOPPAC® freeze compared with market-standard glass vials.
CONCLUSION
In conclusion, there are a number of considerations when selecting the most appropriate PFS for a deep-cold drug. Analysis of the requirements for mRNA-based vaccines showed several reasons why the SCHOTT TOPPAC® freeze syringe is ideally suited for deep-cold temperature drug storage:
- Plunger movement does not breach the PFS sterile barrier, but understanding the syringe and fill-and-finishing parameters helps to control this phenomenon
- COC syringes can maintain container closure integrity down to -100°C because the thermal expansion coefficients of the different syringe components are similar.
- Cross-linked siliconisation reduces the risk for any drug interaction and loss of efficacy by offering superior performance for leachable silicone oil quantities and sub-visible particles
- Normal syringe functionalities are maintained even at storage temperatures down to -100°C
- LNP stability in polymer syringes is comparable with glass vials.
SCHOTT TOPPAC® freeze can therefore be trusted to ensure patient safety in the three key areas of drug stability, syringe functionality and CCI.