To Issue 166
Citation: Hasegawa H, Suzuki T, “OXYCAPT Vial’s Container Closure Integrity with Dry Ice After Freezing and Thawing”. ONdrugDelivery, Issue 166 (Oct 2024), pp 88–93.
Hiroki Hasegawa and Tomohiro Suzuki review the benefits of Mitsubishi Gas Chemical’s multilayer plastic OXYCAPT™ vials and discuss a recent experiment comparing the ability of OXYCAPT and COP vials to resist permeation by CO2 upon thawing after being stored below -60°C, with OXYCAPT’s multilayer structure providing a significant advantage over monolayer COP.
OXYCAPT OVERVIEW
OXYCAPT™ is a multilayer plastic vial and syringe developed by Mitsubishi Gas Chemical (MGC), offering a number of advantageous qualities as a primary drug container, including:
- Excellent oxygen and ultraviolet (UV) light barrier
- Strong water vapour barrier
- Very low extractables
- High pH stability
- Low protein adsorption and aggregation
- High transparency
- High break resistance
- Easy disposability
- Lightweight material.
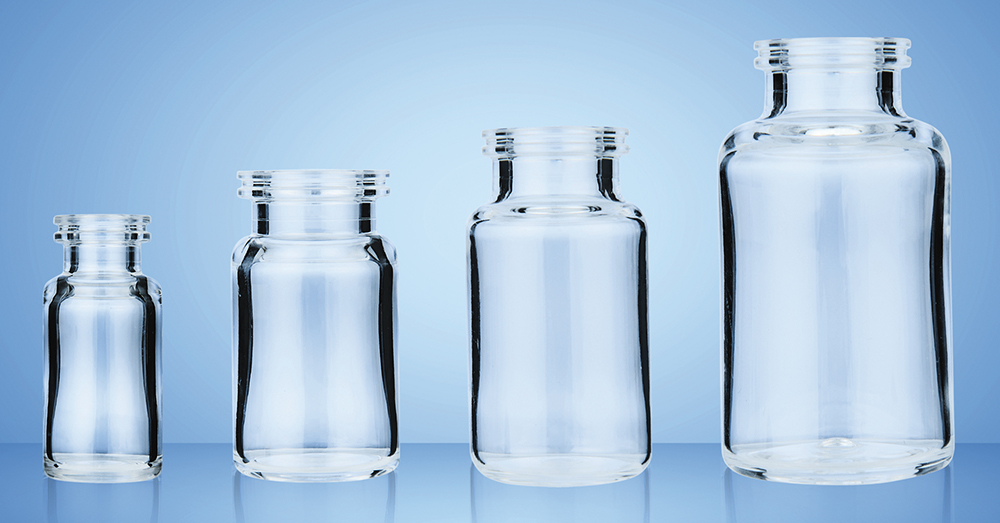
Figure 1: OXYCAPT multilayer plastic vial.
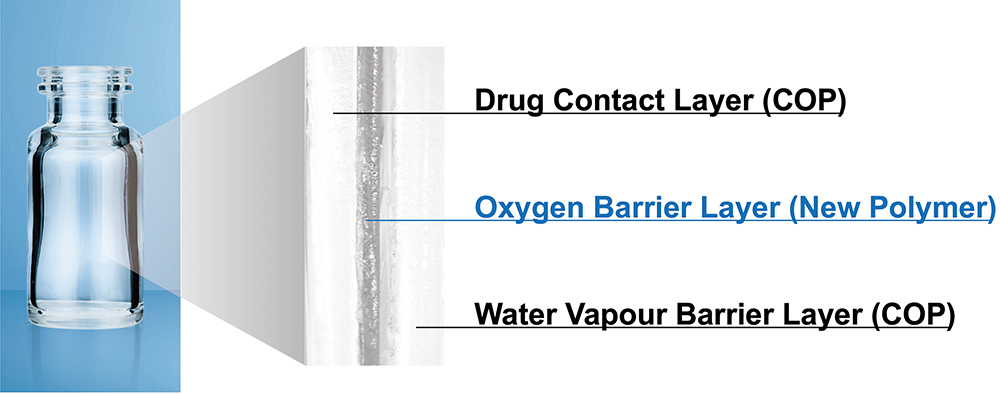
Figure 2: Multilayer structure of OXYCAPT.
MGC continuously conducts studies to confirm these properties. The latest results of these will be shared in the later part of the article. Before that, the first half of this article will provide an overview of the OXYCAPT multilayer plastic vial (Figure 1). The material consists of three layers – the drug contact layer and the outer layer are made of cyclo-olefin polymer (COP) and the oxygen barrier layer is made of MGC’s novel polyester (Figure 2).
MGC recently obtained a report on the environmental impact of glass and plastic containers for medical use from a Japanese research company. The report shows that plastic containers for medical use are much more environmentally friendly compared with glass containers. For example, the carbon footprint, nitrogen oxides emissions, sulfur oxides emissions and water consumption associated with plastic containers for medical use are several times smaller than those of their glass equivalents.
OXYCAPT provides an excellent oxygen barrier. For example, the oxygen barrier of an OXYCAPT vial is about 20 times better than that of a COP monolayer vial. Furthermore, OXYCAPT provides an excellent UV barrier. While about 70% of 300 nm UV light transmits through glass and COP, only 1.7% transmits through OXYCAPT. MGC has confirmed that this feature contributes to the stability of biologics.
While OXYCAPT cannot reach the performance of glass with respect to acting as a water vapour barrier, its properties are similar to those of COP, which has been used for injectable drugs for a long time. This means that OXYCAPT easily meets the requirements of a water vapour barrier set out by the ICH guidelines.
“A second study confirmed that inorganic extractables levels from OXYCAPT were similar to those from COP, which is well known for being an extremely pure polymer with a better extractables profile than Type 1 glass.”
Studies have shown an extremely low level of extractables from OXYCAPT. One study was conducted to confirm the levels of volatile, semi-volatile and non-volatile impurities from OXYCAPT. Water and four solutions (50% ethanol, sodium chloride, sodium hydroxide and phosphoric acid) were selected, and impurities were measured by gas chromatography mass spectrometry (GC-MS) and liquid chromatography-UV spectroscopy-mass spectrometry (LC-UV-MS) after 70 days at 40°C. Compared with the control, impurities were not detected in the OXYCAPT containers. A second study confirmed that inorganic extractables levels from OXYCAPT were similar to those from COP, which is well known for being an extremely pure polymer with a better extractables profile than Type 1 glass. Lower levels of inorganic extractables are known to contribute to better pH stability in drug products.
The OXYCAPT vial is produced by co-injection blow-moulding technology. MGC has also developed inspection methods for testing the oxygen barrier layer. All the containers are fully inspected by state-of-the-art inspection machinery.
MGC can offer bulk vials and ready-to-use (RTU) vials, with its RTU products provided in standard nest-and-tub or tray formats. The nest and tub are mainly sterilised using gamma rays. There are 2, 6, 10 and 20 mL variants for vials. MGC is willing to provide samples for initial testing free of charge.
Each polymer meets the requirements of US Pharmacopeia (USP) regulations USP <661>, USP <87> and USP <88>, as well as those of the European Pharmacopoeia, and has been filed in the US FDA’s drug master file (DMF). The vials are also compliant with each pharmacopoeia and have been filed in the DMF.
The primary target market for OXYCAPT is the therapeutic application of biologics. As mentioned in ICH Q5C (Stability of Biotechnological/Biological Products), oxidation is one of the causes of protein instability. As such, the oxygen and UV barrier properties of OXYCAPT will definitely contribute to the stability of biologics stored within. Furthermore, some drug developers have recently started evaluating the OXYCAPT vials for their gene and cell therapies; the RTU vial is sterilised by gamma radiation, making it ideal for protein-based drugs.
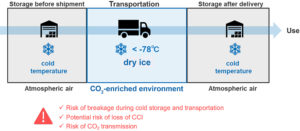
Figure 3: Typical scheme of storage and transportation for gene therapy.
CONTAINER CLOSURE INTEGRITY AT -80°C
All pharmaceutical containers must maintain integrity against microbial contamination and have a gas barrier when a drug is sensitive to oxygen or carbon dioxide (CO2 . Figure 3 shows a typical scheme of storage and transportation for gene therapy. During storage and transportation, packages, including vials, are exposed to temperatures of around -80°C in a deep freezer or dry ice, which is a potential risk to container closure integrity (CCI) due to differences in the coefficient of thermal expansion (CTE) of the vial and rubber closure materials.
“The oxygen barrier of an OXYCAPT vial is about 20 times better than that of a COP monolayer vial.”
The CCI of Type I glass vials is particularly at risk from very low temperatures compared with plastic vials because the CTE of typical Type I glass is a factor of 10 smaller than that of rubber, including halogenated butyl rubber. On the other hand, standard plastic vials have a potential risk of CO2 transmission when in storage with dry ice. Based on MGC’s calculation by measurement of the transmission rate of CO2 through a polymer film, OXYCAPT has a CO2 barrier more than 20 times better than comparable COP monolayer vials. This means that the OXYCAPT vial has the potential to significantly contribute to protecting drugs, including biologics and gene and cell therapies, when they are in transport with dry ice.
To examine this potential benefit further, MGC performed a CCI test with dry ice. Table 1 shows the test sample combinations of OXYCAPT vial and rubber closures. Rubber closure 1 is a typical closure made of bromo butyl rubber with a glass transition temperature of -65°C. MGC also prepared press-on-cap closures and OXYCAPT’s positive control with a fine hole of a 5 μm nominal diameter.
Entry | Vial Configuration | Vial | Rubber Closure | Aluminium seal cap |
1 | OXYCAPT/Rubber closure 1 | OXYCAPT-P 10 mL vial | Bromo butyl rubber | Standard one with closure 1 |
2 | OXYCAPT/Press-on-cap closure 2 | OXYCAPT-P 10 mL vial | Press-on-cap closure | |
3 | OXYCAPT/Rubber closure 1/ Positive control |
OXYCAPT-P 10 mL vial | Bromo butyl rubber | Standard one with closure 1 |
Table 1: Test sample combinations of OXYCAPT vial and rubber closures.
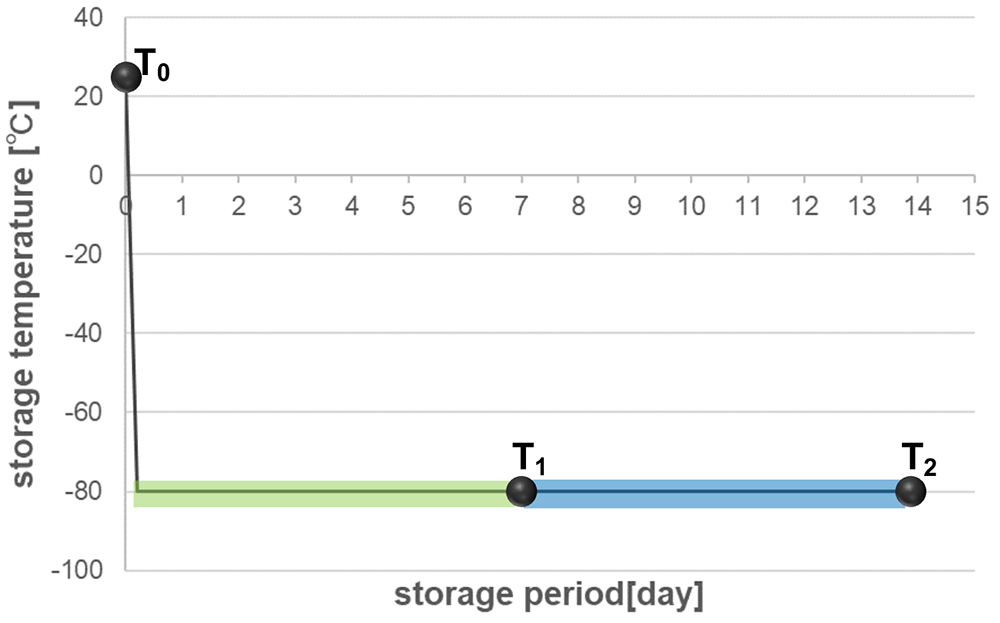
Figure 4: Test procedure of storage in deep freezer and insulation box with dry ice.
Figure 4 shows the test procedure, which includes storage in a deep freezer and an insulation box with dry ice. First, all the vials, closures and aluminium seals were inserted into a chamber where the air was replaced with nitrogen, then they were assembled by hand in the chamber. After preparing the samples, MGC measured the partial pressure of CO2 in the vials’ headspace for all the samples (T0). The samples were then stored in a deep freezer at -80°C for seven days. After storage in the freezer, the CO2 pressure of the headspace was measured (T1). Next, the remaining samples were immediately inserted into an insulation box that was filled with 30 kg of dry ice, as shown in Figure 5. After storage in the CO2 -enriched environment, CO2 pressure in the headspace was measured (T2).
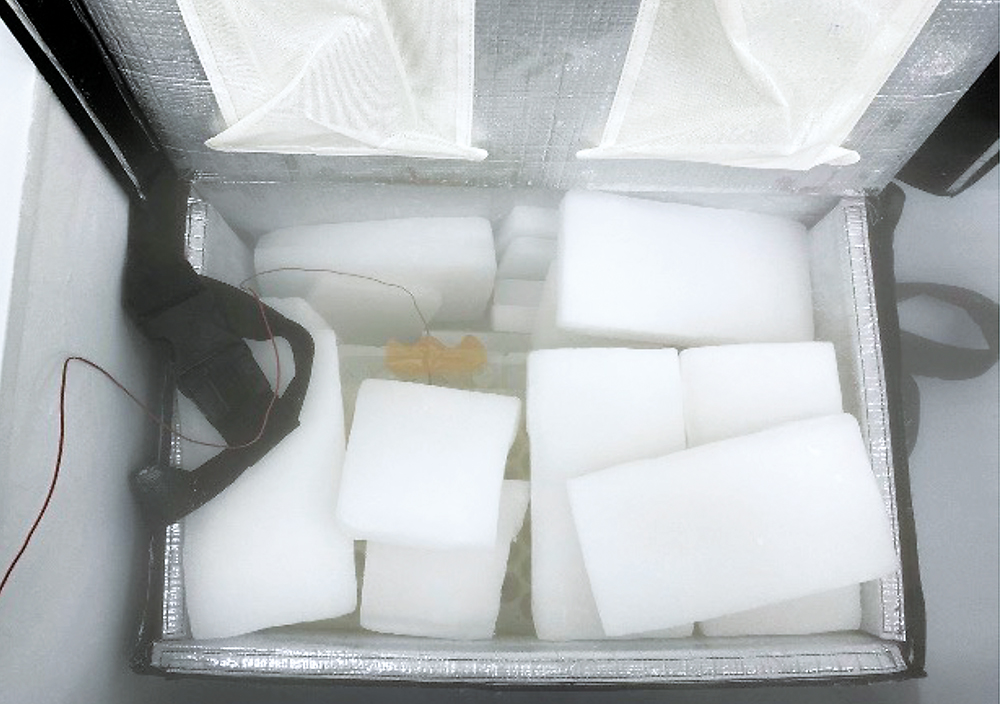
Figure 5: Dry-ice blocks in insulation box.
Headspace pressure of CO2 was measured with an FMS-Carbon Dioxide, manufactured by LIGHTHOUSE Instruments (VA, US). The instrument is based on frequency modulation spectroscopy (FMS), which is a non-destructive method. Table 2 shows the sample number for each measurement time point. The measured vials were disposed of after the measurements at T1 and the remaining ones were measured at T2.
Entry | Vial | Stopper | Number of all Samples | T1 (7 days) |
T2 (7 +7 days) |
1 | OXYCAPT | Rubber closure 1 | 40 | 20 | 20 |
2 | OXYCAPT | Press-on-cap closure 2 | 40 | 20 | 20 |
1′ | OXYCAPT, Positive control | Rubber closure 1 | 10 | 5 | 5 |
Table 2: Sample number for each measurement time point.
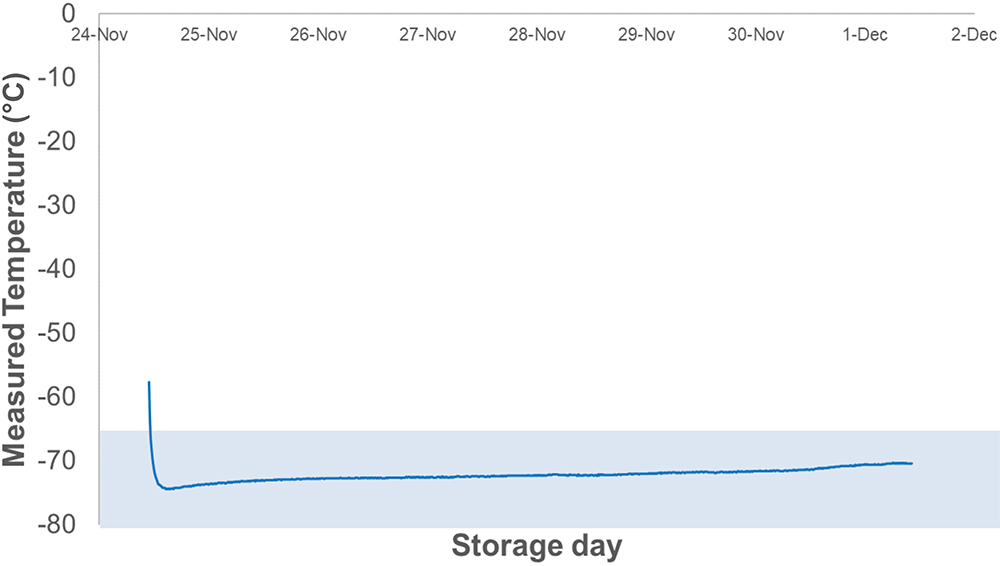
Figure 6: Logging temperature data in the insulation box.
At temperatures lower than -65°C, bromo butyl rubber loses its elastic properties, which may lead to loss of airtightness at the interface between vial and rubber closure. Therefore, maintaining a temperature inside the insulation box of under -65°C is crucial for measuring the leakage precisely in this test. Figure 6 shows a temperature log inside the insulation box during the test, which was kept below -70°C for seven days.
Figure 7 shows the results of headspace CO2 pressure for Entries 1, 2 and 1’. Regarding OXYCAPT positive control of Entry 1’, the mean value of CO2 pressure was 183 Torr at T2 under a CO2 -enriched environment. However, there was no CO2 ingress at T1, as the initial seven-day storage was conducted under atmospheric conditions without dry ice. On the other hand, CO2 ingress was not observed in either combination of OXYCAPT and the two types of closure (Entry 1 and Entry 2), even at T2. This study demonstrated that OXYCAPT has an excellent CCI under a CO2 -enriched environment for seven days.
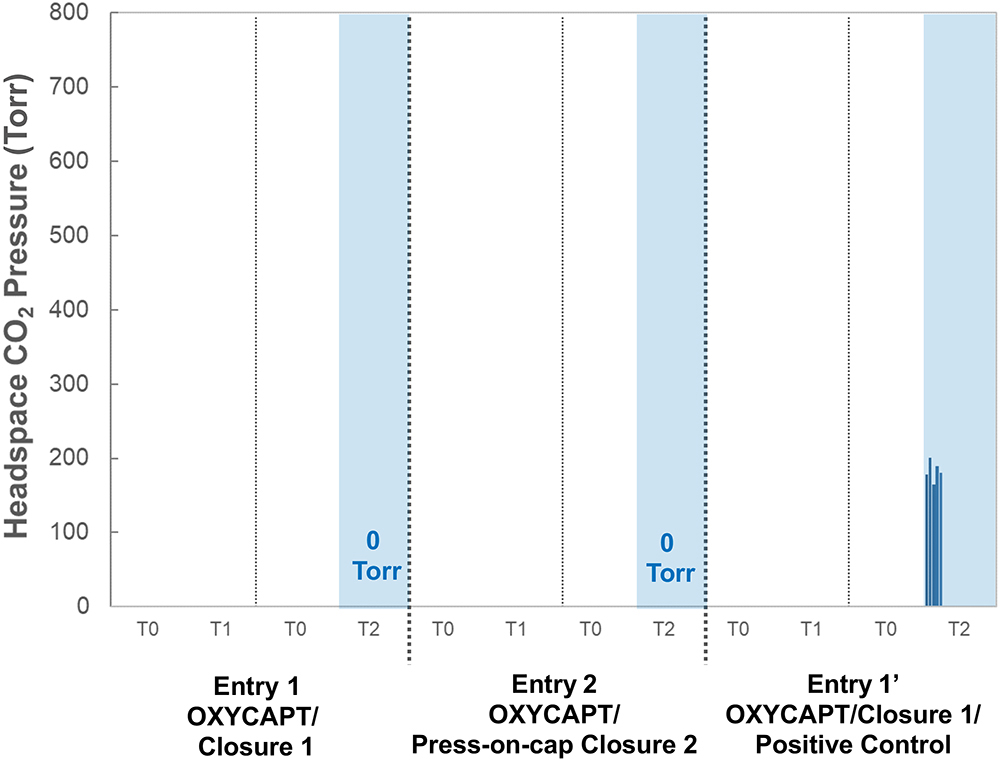
Figure 7: Headspace CO2-enriched pressure for Entry 1, 2 and 1’.
There are several factors that can affect CCI for a combination of vials and closures, including capping force and type of closure, among others. In addition, CO2 transmission is potentially observed in long-term storage with dry ice and an increase in temperature during storage. MGC intends to devise and perform additional CCI tests to clarify the efficiency of OXYCAPT vials compared with other plastic and glass vials. Furthermore, MGC is also planning to conduct similar studies at -180°C to confirm the effectiveness for gene and cell therapies.
CO2 BARRIER OF OXYCAPT
CO2 molecules can permeate through polymers and get into a vial’s headspace, affecting drug stability. As the rate of CO2 transmission is different between polymer materials, MGC performed some related studies using OXYCAPT and COP vials. OXYCAPT and commercially available COP 10R vials were prepared with bromo butyl rubber (BBR) and aluminium seal closures. Firstly, all the vials and BBR and aluminium seals were placed in a nitrogen chamber for a couple of days. Secondly, the vials were sealed with the closures using a hand-crimper in a nitrogen chamber. Thirdly, these vials, filled with nitrogen gas, were placed in a box filled with CO2 and stored at 23°C.
Figure 8 shows the results of headspace CO2 partial pressure of OXYCAPT and COP vials. Although the CO2 partial pressure of COP vials immediately rose, reaching around 700 Torr in 60 days, the OXYCAPT vials were able to keep CO2 partial pressure to very low levels.
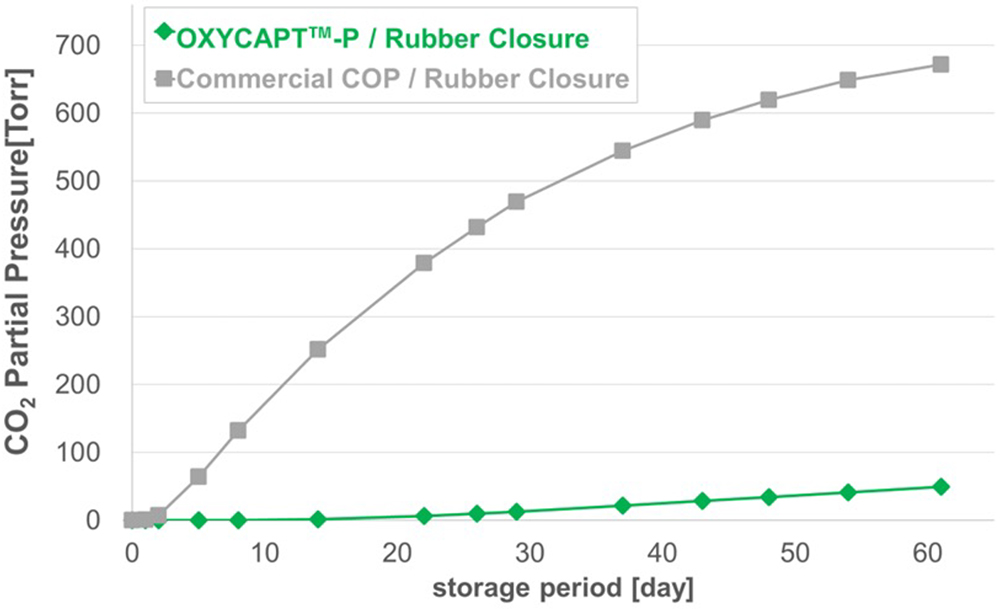
Figure 8: Headspace CO2 partial pressure of OXYCAPT and COP vials.
MGC also calculated the CO2 transmission rate of OXYCAPT and COP vials by using the test results of CO2 partial pressure. While only 0.018 cm3 of CO2 transmitted through OXYCAPT 10R vials per day at 23°C, 0.423 cm3 transmitted through 10R COP vials (Figure 9). This result demonstrates that the CO2 barrier of OXYCAPT is more than 20 times better than that of standard COP.
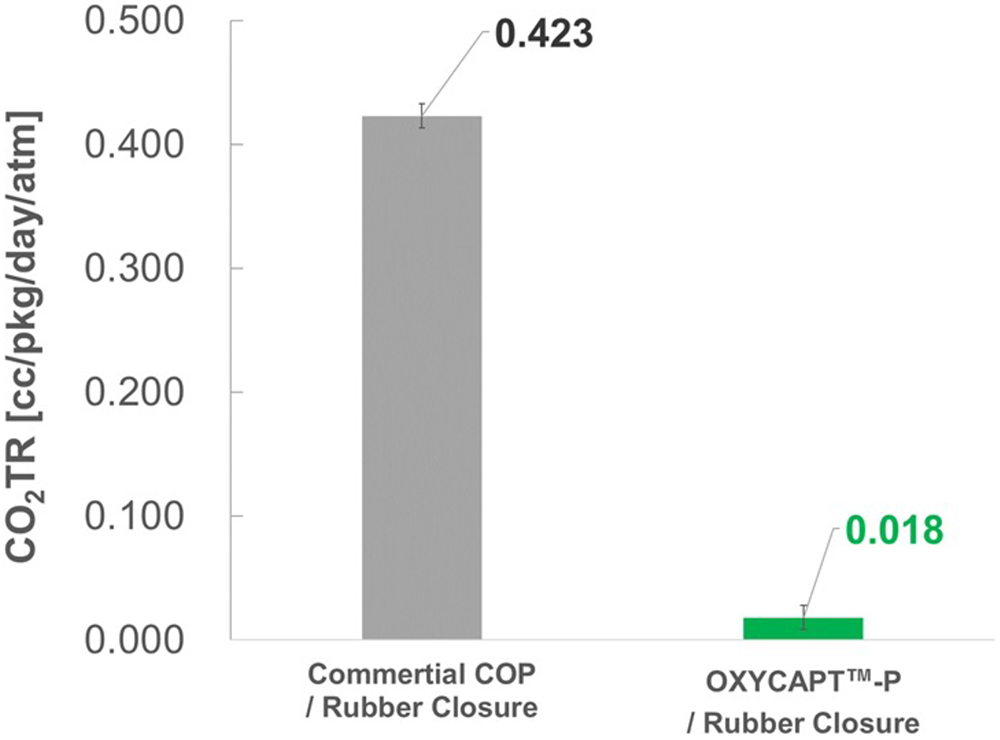
Figure 9: CO2 transmission rate of OXYCAPT and COP vials.
CO2 DIFFUSION UPON THAWING
Theoretically, the phenomenon of gas permeation depends on several physical factors, including the surrounding temperature and permeability coefficient of gas molecules. In accordance with Arrhenius’s formula, gas permeation gets slower as the surrounding temperature becomes lower, meaning that CO2 is barely able to permeate plastic vials into the headspace for the duration of transport with dry ice at lower than -60°C temperatures.
However, there is still a risk of CO2 ingress into the headspace after thawing because there are two steps in CO2 permeation – dissolution and diffusion of CO2 molecules. Dissolution refers to gas molecules being absorbed into the structure of plastics, while diffusion refers to the dissolved gases passing through them. In theory, the permeability coefficient is obtained by multiplying the solubility coefficient with the diffusion coefficient. The diffusion coefficient has a positive correlation with temperature, whereas the solubility coefficient has a negative correlation to it in the case of CO2 . In other words, plastic vials are likely to be able to retain CO2 within their structure during transport with dry ice below -60°C, which can then cause CO2 ingress after the thawing process (Figure 10).
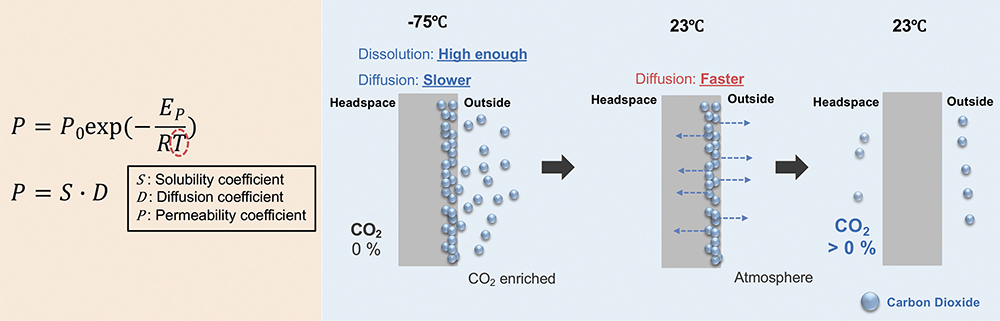
Figure 10: Process of gas permeation.
To verify this theory, MGC conducted a study on CO2 ingress after freezing and thawing. To imitate the typical storage and transport process for gene therapy products, MGC first stored OXYCAPT and COP vials at -75°C in a CO2 -rich environment for 18 days and then placed them at room temperature under atmospheric conditions for an additional four days.
“OXYCAPT was able to prevent the CO2 diffusion into the headspace upon thawing due to its highly effective CO2 barrier layer, which dramatically mitigated any permeation of the CO2 dissolved in the outer layer of the multilayer.”
As MGC expected, both the COP and OXYCAPT vials had 0% CO2 in the headspace after storage at -75°C in a CO2 -rich environment for 18 days. However, the COP vial could not maintain 0% CO2 after the additional time under atmospheric conditions, whereas OXYCAPT was able to sustain 0% CO2 (Figure 11). It is presumed that the dissolved CO2 diffused through the monolayer COP vials when thawed to room temperature. On the other hand, OXYCAPT was able to prevent CO2 diffusion into the headspace upon thawing due to its highly effective CO2 barrier layer, which dramatically mitigated any permeation of the CO2 dissolved in the outer layer of the multilayer.
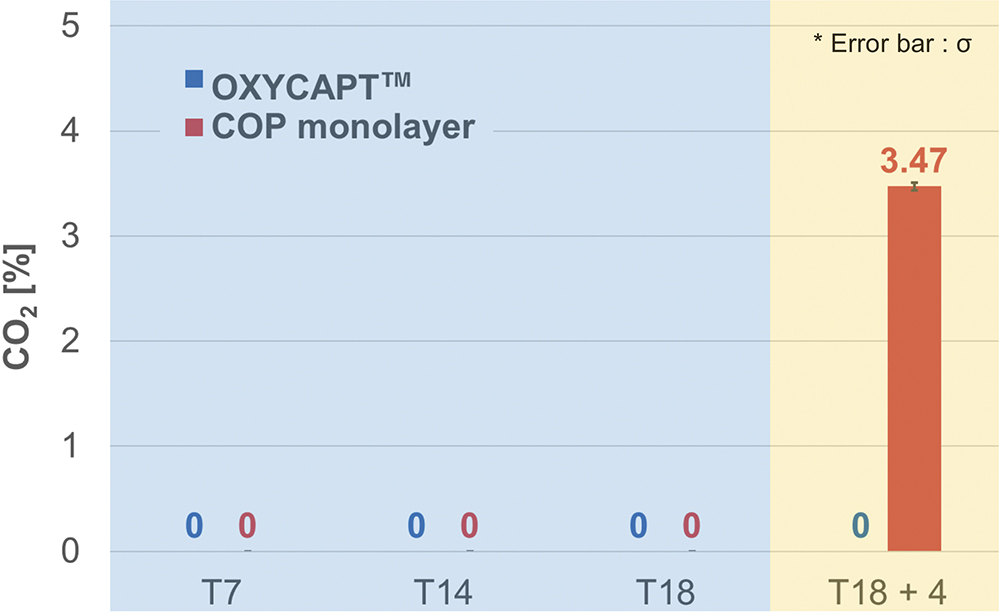
Figure 11: CO2 transmission rate of OXYCAPT and COP vials.
To verify the excellent properties of OXYCAPT for cell gene therapy products, MGC has started further studies, such as CCI testing with liquid nitrogen and pH shift with dry ice. Although it is said that glass cannot be used for the cell gene therapy products stored at deep-cold or cryogenic temperatures, MGC has also added Type 1 glass vials to the samples to confirm if this is true or false.
CONCLUSION
These latest results have contributed to the ongoing studies verifying OXYCAPT’s superior properties for biologics and gene and cell therapies. In addition to the advantages of COP, such as a strong water vapour barrier, high break resistance, very low extractables and low protein adsorption, OXYCAPT also provides strong oxygen and UV light barriers. In particular, the excellent CO2 barrier of OXYCAPT is very useful for the stability of gene and cell therapies stored with dry ice. MGC believes that OXYCAPT offers a multitude of benefits to the rapidly growing field of biologics and gene and cell therapies.
Previous article
ROUNDTABLE: DISCUSSION ON ANTI-OBESITY TREATMENTSNext article
NOLATO’S VIRTUAL FACTORY