To Issue 156
Citation: Yeh H-J, Lai J-R, Cheng T-C, “Advancing Subcutaneous Delivery of Biologics: A Novel Reusable Mems-Enabled Actuator”. ONdrugDelivery, Issue 156 (Jan 2024), pp 117–126.
Hong-Jun Yeh, Jiunn-Ru Lai and Tsung-Chieh Cheng explore the challenges and opportunities related to the subcutaneous delivery of biotherapeutics. They also highlight a novel reusable MEMS-enabled pneumatic actuation system designed to overcome these challenges.
The biologics drug market continues to grow rapidly, with subcutaneous (SC) administration becoming increasingly popular due to health-economic reasons and advancements in delivery technologies. Biologic therapeutics have transformed the ability to effectively treat diseases in areas such as oncology, inflammation, diabetes and cardiovascular, immunological and genetic/metabolic disorders. Unlike small-molecule drugs (oral tablets), biomacromolecules have the unique ability to bind with targeted cells during therapeutic treatment and prevent unwanted drug-to-drug interactions. However, rapid enzymatic degradation and poor absorption in the harsh environment of the gastrointestinal tract limit its administration to parenteral (mainly injectable) routes.
The discovery and development efforts of biologic therapeutics have been the focus of the biopharma industry in recent decades. As a result, the global biologics market size was valued at US$512 billion (£405 billion) in 2023 and is projected to grow at a compound annual growth rate of 10.3% from 2023 to 2030, reaching $1,009 billion.1
One major active area in terms of new biomacromolecule development in recent years is high-concentration antibodies, such as monoclonal antibodies (mAbs). Since their introduction as therapeutic drugs, mAbs therapies have enjoyed tremendous commercial success with continuous growing opportunities. Due to their success in treatment over the past two decades, the global therapeutic market for mAbs is projected to grow to about $300 billion by 2025.2 As of 2023, mAbs are also the most marketed biologic, with over 4,000 mAbs undergoing preclinical or clinical development.3 One key reason for the success of mAbs in the therapeutic sector is SC self-administration, which also helped expedite the advancement of biologics formulation and device functionality.
Injectable routes serve as alternative approaches for drug administration, distinct from the oral/digestive route. The injectable drugs market is projected to witness continued growth, with an expected market valuation of $937 billion by 2032.4 Intravenous (IV) and SC routes are the primary administration routes within the injectable market. However, in recent years, biologics delivery has shifted noticeably from IV to SC. This trend is driven by both health-economic factors and advancements in SC drug delivery technologies.
Spurred by technological advancements addressing the demand for high-dose delivery of biotherapeutics, emerging trends in SC drug delivery can be largely categorised into two areas. Firstly, novel formulations enable the development of higher-concentration biotherapeutics (>200 mg/mL) while also mitigating concerns related to aggregation and inherent immunogenicity. Another key formulation advancement is the incorporation of the dispersion enhancer recombinant human hyaluronidase (rHuPH20) to enhance the absorption of injection volumes in the SC space, while maintaining drug stability throughout their target shelf life.5,6,7
Secondly, novel device technologies facilitate the delivery of larger doses through increased volumes and/or concentration. These technologies including autoinjectors with 5 to 10 mL volume, on-body injectors/on-body delivery systems (OBIs/OBDSs) with 20 to 50 mL volume, and ambulatory infusion pumps with 10 to >250 mL volume, are all capable of handling higher drug concentrations of 120 mg/mL or above subcutaneously. Although many of these device technologies are still in development and require clinical validation and eventual approval for commercial use, the SC route of administration is expected to continue its growth trajectory in the foreseeable future.
Previously, MicroMED presented two technical papers in ONdrugDelivery (here and here) to discuss the technical data and applications of the single-use microelectromechanical system (MEMS)-enabled actuator.8,9 This article aims to provide a comprehensive review and discussion of the current landscape of the SC drug delivery system (DDS) for biotherapeutics. It will specifically focus on examining the challenges and opportunities associated with these systems, emphasising new device functionalities and capabilities. The primary focus is on device functionality aspects, therefore, the article will not take physiological and pharmacokinetic (PK) factors into consideration to avoid unnecessary complexity.
With a focus on emerging opportunities, this article introduces MicroMED’s innovative reusable MEMS-enabled actuator. It presents its working principles, supported by the functional test data, and conducts a comparative analysis with other DDSs using existing drivers to demonstrate how the novel actuator is capable of fulfilling the unmet needs resulting from emerging trends in the overall DDS market.
SHIFTING FROM IV TO SC
Compared with IV, SC delivery of biologics eliminates the need for frequent hospital visits, reduces the risk of infections and infusion-related reactions, and provides a more convenient and flexible treatment option.10 Successful examples of introducing the SC route for biologics delivery after IV route approval have established compelling cases for the SC route due to health-economic advantages. These are some of the reasons why the SC route of administration is being considered more often than IV when dealing with formulation, stability evaluation and final commercialisation. SC applications can also serve as a lifecycle management strategy for a particular indication when competition is a consideration. The following are key reasons why cases of shifting from IV to SC will continue to increase:
- Easier dosing for SC than IV
- Fixed-dose regimen versus complicated bodyweight-adjusted dosing
- SC is easier to handle for combination therapy11,12
- Convenience: self-administration at-home setting
- Increased compliance due to patient convenience and preference
- Cost and time saving – fewer healthcare professionals, facilities and resources required
- Challenges posed by situations like covid-19 or any other pandemic, making travel for IV treatment difficult
- Remote monitoring due to Internet of Things availability
- Higher doses are possible through SC formulation advances, such as high-concentration or higher-volume SC injections by adding dispersion/absorption enhancers (sequential injection) or co-administration with rHuPH20.
Some existing examples of converting from IV to SC routes that have achieved both technical and commercial success include daratumumab, rituximab, adalimumab and trastuzumab.
OVERALL LANDSCAPE OF SC BIOLOGICS DELIVERY SYSTEMS AND NEW TRENDS
The overall SC DDS market for biologics is influenced by various factors, including the following:
- Continued growth of the biotherapeutic market
- Shift from IV to SC administration
- Maintenance of the competitive edge due to devices with similar indications and biosimilars
- Cost reduction
- Device and drug lifecycle management
- New DDS functionalities/designs to meet emerging trends and requirements.
New functionality and design considerations:
- Unique biologics formulations that require specific concentration, volume and flow rate
- Reusability (multiple injections) and recyclability (components) to address sustainability needs
- Flexible and accurate control of dosage and flow rate
- Human factors engineering (HFE), tolerability, human-machine interface (HMI) with connectivity, and digitisation to improve patient compliance and safety
- Non-traditional actuation methods for unique applications without using traditional primary containers like prefilled syringes (PFSs) or cartridges
- Addressing issues associated with traditional SC DDSs that use spring, motor, elastic material or gas cylinder-driven mechanisms.
Figure 1 illustrates the current landscape of the SC biologics DDS market.
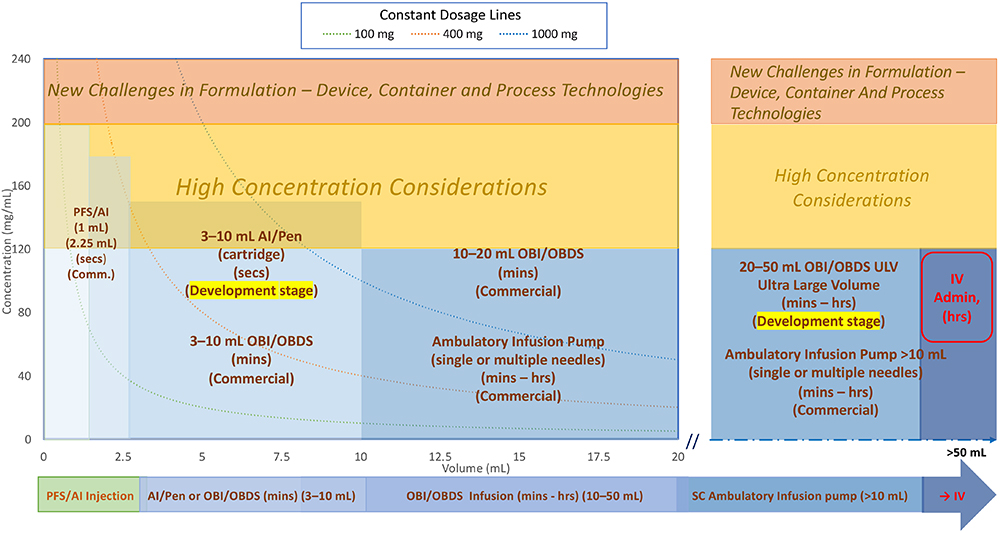
Figure 1: Overall SC delivery device design space/landscape.
Two main points to consider when reviewing the DDS landscape:
- Formulation-related factors including concentration, volume and dosage:
a. Formulation stability evaluation, encompassing manufacturing, storage, transportation and drug delivery processes.
b. Volume, concentration and injection/infusion duration are typically considered together, determining whether a small volume at high concentration with short duration (mainly for autoinjector/pen) or a high volume at low concentration or high volume at high concentration with short or long duration (mainly for OBI or infusion pump) is preferred for the same dose. These are the main consideration factors for device selection.
c. Concentrations >120–200 mg/mL pose potential formulation development challenges and risks.
d. Concentrations exceeding 200 mg/mL are considered difficult to develop and require careful consideration.13
e. Constant dosage lines at 100, 400 and 1,000 mg are represented by these three curve lines (typical trend is from upper left to lower right).
f. Higher-volume formulations may leverage dispersion/absorption enhancers with rHuPH20. Either pre-injecting rHuPH20 followed by the injection of therapeutic biologics or incorporating rHuPH20 into the formulation development can enhance permeation in the SC space.
g. Additional considerations needed for fixed-dose combination therapies requirements. - Human factor and tolerability considerations affecting formulation and device design:
a. Pain and sensation associated with injection or infusion, may be influenced by factors such as viscosity, volume, speed of injection, needle size and formulation
b. Injection or infusion duration, varying from seconds to minutes or hours
c. Frequency of injection or infusion
d. Patient skin reactions, such as swelling, wheal formation and erythema
e. Impact on quality of life, evaluating injection time length, frequency, wearable (adhesive) or portable (belt, strap) options
f. Usability enhancements through HMI, connectivity (built-in or add-on) and digitisation to improve patient compliance and safety.
Review of the Current DDS Market Landscape
As indicated in Figure 1, the existing DDSs can be categorised into three main groups. These categories provide an overview of the current landscape of SC biologics DDSs. By considering formulation-related factors and human factor/tolerability aspects, manufacturers can design and develop effective and patient-friendly delivery systems.
- PFS with needle safety device, autoinjectors and pen injectors (left-hand side of Figure 1):
a. This category includes 1 and 2.25 mL PFS and autoinjector devices with a wide range of concentrations.
b. Dose volumes of 1 and 2.25 mL are commercially available for PFSs, autoinjectors and pen injectors.
c. Larger-volume autoinjectors (5–10 mL),14 (cartridge-based) are available for development studies but not yet commercially available.
d. Key characteristics include short injection times (<30 seconds)15 to meet human factors and usability requirements, although more clinical trials and regulatory confirmation are needed.
e. Short injection time requirements limit volumes.
f. Higher concentrations (120 mg/mL or higher) are often needed to achieve the desired dosage due to volume limitations. They require more careful stability evaluation, such as aggregation and immunogenicity.
g. Formulations for larger-volume devices (e.g. 5–10 mL) may incorporate dispersion/absorption enhancers with rHuPH20.
h. Limited number of reusable autoinjectors/pens are commercially available.
i. Spring, gas cylinder and motor-driven autoinjectors/pen injectors are available, but they all have pros and cons. The key challenges are the handling of high concentration (high viscosity) where you have to consider sufficient force output from the drivers and glass container breakage due to high initial impact. - Wearable OBIs, OBDSs or large- volume injectors:
a. Dose volumes range from 3 mL to 50 mL, although commercially available DDSs are limited to the 3–20 mL range. The upper limit for on-body wearable devices is typically 50 mL due to size and weight limitations
b. Injection or infusion times can vary from minutes to hours, depending on volume and dosing regimen requirements
c. Drive mechanism – mechanical (spring-based or elastomeric tube-based) and motor-based electromechanical OBIs are available.
d. Primary containers include PFSs, cartridges and custom-made special containers
e. Vial or PFS transfer units are available when special containers are used
f. The needle insertion unit includes a soft tube that remains in the SC tissue for the duration of the injection or infusion
g. Manual connections with infusion sets can be designed for single or multiple needle site infusion sets instead of the needle insertion unit. - Infusion pumps (ambulatory) (mainly on the right-hand side of Figure 1):
a. Dose volumes range from 10 mL to over 250 mL and can be wearable/portable using a belt or strap
b. Flow rate for infusion pumps tends to be on the lower side due to larger volume and longer infusion duration. This helps to accommodate infusion of drugs with higher concentration
c. Spring-driven mechanical pumps are typically larger in size, use standard disposable syringes and are equipped with some flow-rate controls. Their typical cost is lower compared with motor driven ones
d. Elastomeric container-driven mechanical pumps are also available for low-cost options with less flow accuracy
e. Motor-driven electromechanical pumps with more user settings and accurate controls are available. Some pumps need custom-made syringes, and they are typically reusable but more expensive
f. Infusion sets with flow-rate control allow for single or multiple needle site infusion (high total infusion rate)
g. Higher-energy driving mechanisms compared with OBI devices are needed due to the extra flow resistance from the long tubing of the infusion set.
Emerging Trends in SC Biologics DDS
To ensure effective treatment, higher doses of biotherapeutics are crucial, leading to the development of new technologies that address formulation and device functionality. On the formulation side, advancements in higher-concentration formulations and formulations incorporating absorption/dispersion enhancer rHuPH20 offer additional solutions for delivering high doses of biotherapeutics through various DDSs.
New Trends for Autoinjectors and Pen Injectors
- Expanded range of volume selection: 3–10 mL
- Wide range of viscosity selection: 1–100 cP
- Short delivery time: <30 seconds at 10 mL
- Faster flow rate range: 0.06–0.9 mL/s
- Use of rHuPH20 for high volumes (>3 mL)
- Reusability for sustainability
- Usability enhancements through HMI, connectivity and digitisation to improve patient compliance and safety.
Higher-concentration formulations enable high-dose delivery in small volumes, particularly for autoinjector and pen injector applications requiring short injection times (<30 seconds). When larger volumes (5–10 mL) are needed for autoinjector and pen injector applications, pre-injecting rHuPH20 followed by the injection of therapeutic biologics or incorporating it into the formulation can enhance permeation in the SC space. However, clinical and regulatory confirmation for autoinjector/pen injection devices with volumes exceeding 3 mL is necessary for commercial use. Currently, few commercially available autoinjector/pen devices feature reusable drive units and disposable container designs, but sustainability efforts aiming to minimise material waste have become essential. Connectivity features, including digitisation, can be built in or added as an optional feature for DDSs.
New Trends for Wearable OBIs
- Expanded range of volume selection: 3–50 mL (limited to 50 mL due to drug and device weight and size considerations for patient convenience)
- Wide range of viscosity selection: 1–80 cP
- Wide range of injection/infusion time selection: minutes to hours
- Flow rate range: 0.01–0.07 mL/s or 0.6–4 mL/min
- Option for using rHuPH20
- Exploring new driving mechanisms beyond the traditional linear actuation for space efficiency with flow control
- Single and multiple needle sites with infusion set connection for large volumes
- Solution transfer options: direct transfer from PFS or vial
- Reusability for sustainability
- Usability enhancements through HMI, connectivity and digitisation to improve patient compliance and safety.
SC OBIs and OBDSs address the need for larger-volume applications (3–50 mL). They are driven by competition in the biosimilars market and formulation challenges of higher-concentration biologics required for autoinjectors are limited to dose volumes of 1 and 2.25 mL. Currently, only a limited number of OBI/OBDS devices with volume between 3 and 20 mL are commercially available but all lack reusable drive designs. Many OBI/OBDS devices with different drivers, design approaches and specifications are still in the development stage. Motor-driven OBIs/OBDSs are mainstream in this category due to their design flexibility. But drawbacks like too many moving parts required are making higher-volume (>20 mL) OBIs/OBDSs very challenging.
New Trends for Wearable Infusion Pumps (Ambulatory)
- Expanded range of volume selection: 10 to >250 mL (patient convenience considerations)
- Wide range of viscosity selection: 1–50 cP
- Expanded range of infusion time selection: minutes to long hours
- Slower flow rate range: 0.01–0.04 mL/s or 0.6–2.5 mL/min
- Single and multiple injection/needle sites with infusion set connection
- Adjustable flow rate
- Option for using rHuPH20
- Exploring new drive mechanisms beyond the traditional linear actuation for space efficiency with accurate flow control
- Exploring better design to address rapid push SC immunoglobulin (SCIg)
- Reusability for sustainability
- Usability enhancements through HMI, connectivity and digitisation to improve patient compliance.
Infusion pumps have been used for IV delivery applications for many years. However, there is a shift towards SC delivery for infusion pump applications, such as IV immunoglobulin (IVIg) and SCIg, which are available for Ig replacement therapy for primary immunodeficiency and chronic inflammatory demyelinating polyneuropathy.16 SCIg offers advantages such as simpler operation and shorter infusion durations.
With advancements in formulation and device technologies for larger-volume (10 to >250 mL) SC delivery, there is a need to reassess the functionalities of existing ambulatory infusion pumps to meet specific SC delivery requirements, including:
- Higher flow rates (shorter total delivery time), higher drug concentrations and volume (leveraging rHuPH20) necessitating larger driving forces
- Rapid push SCIg has gained more popularity than conventional pump infusion with shorter injection time and slightly more frequent injections17
- Patient convenience, achieved through pump size reduction for wearable/portable devices and ease of self-administration
- Improved convenience in terms of handling solution transfer from containers that are typically used for SC injection including lyophilised products.
Summary of the Unmet Emerging Trends for SC DDS – New and Expanding Requirements
The following high-level trends address the unmet needs and expanding requirements in SC DDSs for autoinjectors/pen injectors, OBIs/OBDSs and infusion pumps:
- Adaptable drive platform to address wider volume, concentration and flow rate range
- Scalable size based on different demands and applications
- Flexible, simple and reliable in terms of design for manufacturing and assembly
- Reusable and low energy consumption drive unit to reduce cost and address sustainability concerns
- Flexible and accurate control of dosing regimen (dosage and flow rate)
- Usability enhancement through HMI, connectivity and digitisation for patient compliance and safety
- Non-traditional actuation methods for unique applications without traditional primary containers
- Addressing performance issues and design limitations of traditional spring, motor, gas cylinder and elastomeric container driven DDSs.
NEW REUSABLE MEMS-ENABLED PNEUMATIC ACTUATOR ADDRESSING EMERGING TRENDS OF SC DELIVERY OF BIOLOGICS
As indicated in the previous summary, there is a need for a better and versatile drive mechanism platform for the DDS market that can meet the ever-evolving new requirements coming from the emerging trends. As a start-up medtech company, MicroMED has a patented MEMS-based core technology that offers a perfect drive mechanism platform for flexible DDSs focusing on – but not limited to – the SC route.
Working Principles
The MEMS-enabled actuator is a gas micropump-based system that offers a simple, small size, robust and powerful solution to address emerging unmet needs in drug delivery. The actuator leverages the unique capabilities of MEMS technology to provide a highly efficient and precise solution for SC drug delivery. MEMS technology enables the integration of mechanical and electrical components on a microscopic scale, allowing for miniaturised and high-performance systems.
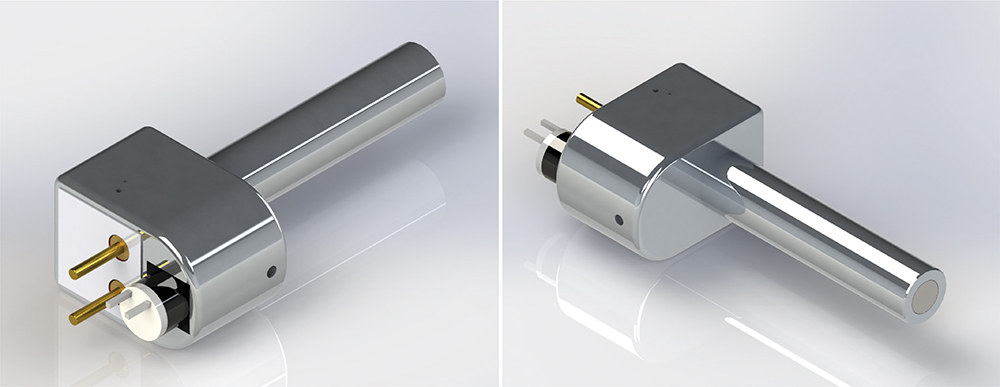
Figure 2: Reusable MEMS-enabled actuation system – MicroMED Primary Actuator®.
Figure 2 illustrates the initial version of the form factor for the MEMS-enabled actuator. With the simplicity and few components needed to integrate the actuator into a final delivery device, MicroMED has the flexibility to adjust the form factor based on the requirements of the final device products.
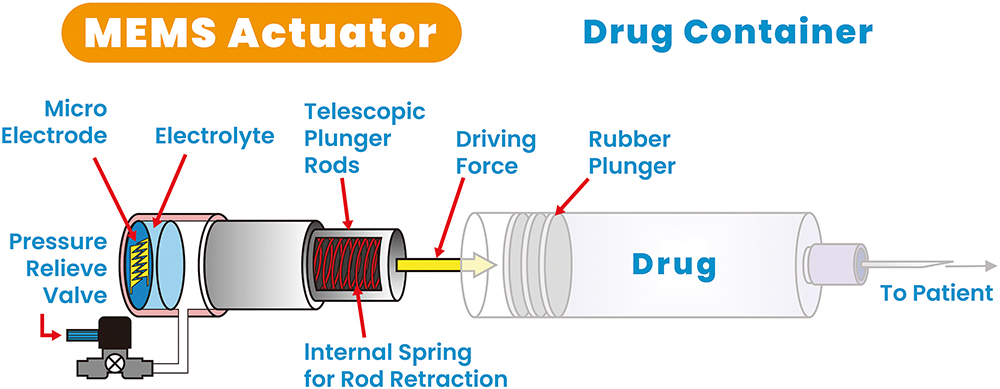
Figure 3: Reusable MEMS-enabled actuator – key components.
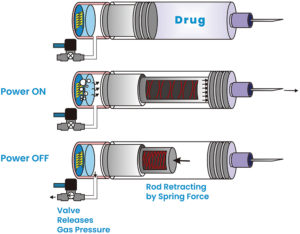
Figure 4: Operation steps.
As indicated in Figures 3 and 4, the actuator is a gas micropump that employs MEMS technology and electrochemical principles to convert electrolyte into gas pressure in a fast and controlled manner. In the actuator, the MEMS microelectrodes, controlled by a printed circuit board assembly, interact with the electrolyte to initiate instantaneous gas generation. This precise control over gas generation enables accurate gas pressure output, resulting in a force output from the telescopic plunger rod. The plunger rod pushes the plunger stopper forwards until it reaches the end of the syringe barrel, completing a single injection cycle as indicated in the middle diagram of Figure 4.
Figure 4 shows the key steps of the actuator operation. After the injection, when the power is turned off, the valve opens and releases the gas pressure. The stretched spring then initiates the retraction of the plunger rod, preparing it for the next injection cycle. The actuator’s design allows for a high conversion ratio of electrolyte volume to gas volume, typically 1:1,000. This means that a single load of 1 mL of electrolyte can generate 1,000 mL of gas volume, enabling multiple injections with a single load of electrolyte.
Table 1 shows the existing product specifications for the primary actuator, including the new reusable actuators.
Main Application | 10 mL OBI | 2.25 mL AI | 5 mL AI | 50 mL OBI/Infusion Pump |
Model | PA-J Series | PA-E Series | PA-H Series | – |
Container | Cartridge | PFS | PFS | Custom Syringe |
ISO Certified | Yes | On-going | On-going | – |
Single-use/Reuse | Single | Reuse | Single | Reuse |
Injection Volume | 10 mL | 2.25 mL | 5 mL | 50 mL |
Max Input Voltage (VDC) | 7.5 V | 10.5 V | 10.5 V | 7.5 V |
Max Input Power | 15.0 W | 31.5 W | 31.5 W | 15.0 W |
OP. CONDITIONS: Voltage (VDC) Power Viscosity Needle Size Flow Rate Injection Time |
0.4W 8.5 cP 26G Up to 1 mL/min 10 min |
7.5V 15.0W 20 cP 27GTW Up to 4.5 mL/min 30 sec |
7.5V 15.0W 8.5 cP 26GTW Up to 10 mL/min 30 sec |
5V 1W 15 cP 26G Up to 1 mL/min 50 min |
Stroke | 39 mm | 41 mm | 46 mm | 46 mm |
Maximum Static Force | 1,000 N | 1,000 N | 1,000 N | 1,000 N |
Ingress Protection* | IP66 | – | – | – |
Operating Temperature | 0°C–60°C (32°F–140°F) |
0°C–60°C (32°F–140°F) |
0°C–60°C (32°F–140°F) |
0°C–60°C (32°F–140°F) |
Operating Noise | <30dB | <30dB | <30dB | <30dB |
Pin Length | 11.9 mm | 11.9 mm | 11.9 mm | 11.9 mm |
MATERIALS: Telescopic Rods Actuator Housing Input Pins |
Stainless Steel Polycarbonate Copper |
Aluminum Alloy Aluminum Alloy Copper |
Stainless Steel Polycarbonate Copper |
Aluminum Alloy Aluminum Alloy Copper |
DIMENSION: Housing Body Rod |
23x23x16.3 mm 13(D)x16.3(L)mm |
17x25x16 mm 8.5(D)x38.8(L)mm |
18x18x16.3 mm 1.5(D)x41.8(L) mm |
21x21x11.5 mm 15(D)x53(L) mm |
Table 1: Existing product specifications for the primary actuator®.
* IEC 60529 standard tested.
The actuator demonstrates consistent injection cycles, even without any sophisticated feedback control, as shown in Figure 5. The graph illustrates the results of 36 injections using a 2.25 mL drug-filled PFS with 60% glycerine (~10 cP). With monthly injections, the actuator can provide three years of shelf life for the final delivery device. This is the first batch of MicroMED’s initial reusable actuator design. The company is continuing to optimise the design to further advance the performance of the actuator. Even with the first test batch, performance remains very stable, showcasing its reliability and repeatability.
Additionally, the company is validating its actuator design to ensure the actuator’s recyclability. After completing its first shelf life, the actuator can be recycled, refilled with electrolyte and recalibrated for use in a new DDS. This feature enhances sustainability and cost-effectiveness by extending the lifespan of the actuator.
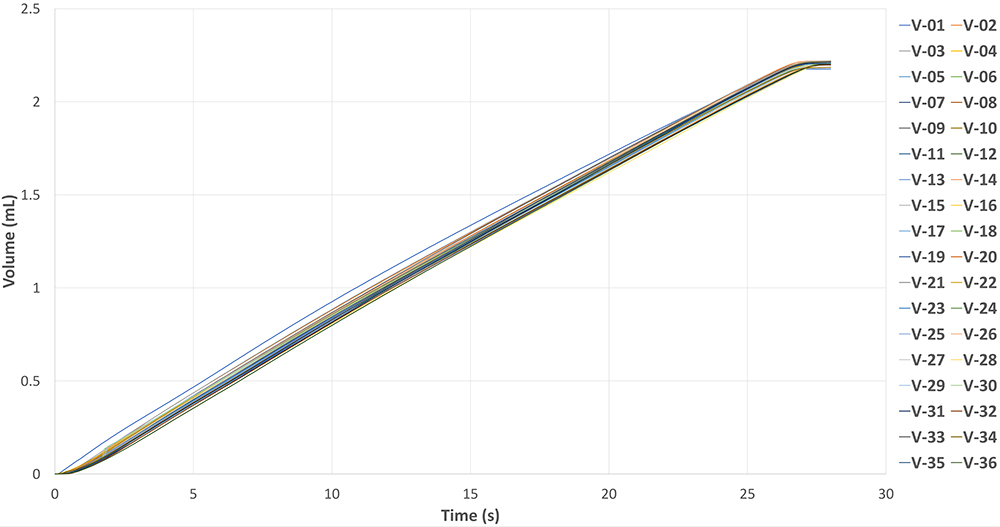
Figure 5: Reusability test data – 2.25 mL 10 cP PFS 27G needle with MEMS-enabled actuator.
The actuator can be configured as a linear actuator, where the gas pressure is transmitted through an enclosed telescopic plunger rod system, or as a non-linear actuator, where the actuation is directly performed by the gas micropump system. This flexibility allows the system to adapt to different drug delivery system designs and requirements.
Why Choose a MEMS-Enabled Actuator?
The following list summarises the advantages of using the MEMS-enabled actuator as a platform for the drive mechanism of different DDS needs:
- Sustainability: reusable per single shelf life and recyclable actuator to address sustainability
- Small but scalable size, low energy consumption and low costs
- Precision control – dose and flow rate
- Low impact but powerful force output
- Adaptable to existing autoinjectors/pen injectors, OBIs/OBDSs, infusion pump DDSs and new unique gas pump applications
- Ability to address high-volume/viscosity biologics delivery
- Linear and nonlinear actuation to address unmet SC delivery needs and other unique applications
- Usability enhancement by integration of HMI and connectivity for digitalisation and patient compliance and safety.
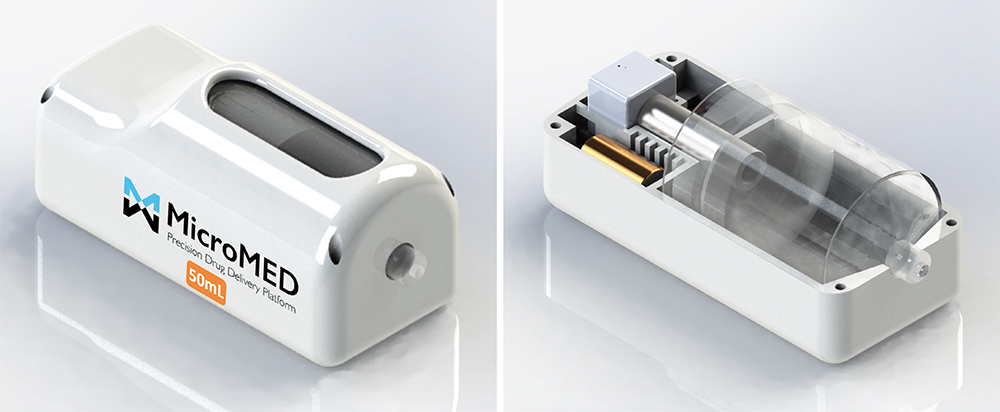
Figure 6: MEMS-actuator-driven 50 mL OBI ambulatory infusion pump – functional prototype.
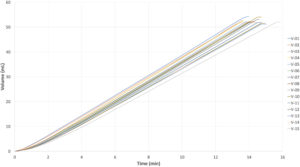
Figure 7: MEMS-actuator-driven ambulatory OBI/infusion pump (functional prototype) – delivers 15 repeatable injections of 50 mL 5 cP, 26G four-needle site infusion set in ~14 mins.
Figure 6 demonstrates the simplicity of integration of a MEMS-enabled actuator into a prototype design of an OBI or ambulatory infusion pump. The layout diagram on the right illustrates the simplicity of adapting or integrating a MEMS actuator into an existing or new DDS. This integration showcases the actuator’s capacity to deliver a 50 mL 5 cP solution using a 26G four-needle site infusion set within approximately 14 minutes with 15 repeated injections, as demonstrated in Figure 7. It is important to note that this test was conducted with a four-needle site infusion set that has a long tubing length of 70 cm, resulting in higher flow resistance compared with the regular fluid path of a typical OBI.
Comparison with Existing DDS Drivers
While no single driver can meet all the attributes and requirements for all DDSs, the MEMS-enabled actuator clearly stands out as the best candidate to address the emerging trends in SC biologics delivery. Table 2 (see over page) highlights the unique advantages of the MEMS-enabled actuator and underscores its suitability for the evolving requirements in SC DDSs.
When compared with existing drivers for SC DDSs, the MEMS-enabled actuator stands out due to its comprehensive capabilities and flexibility to adapt to existing delivery devices and adeptness to become a scalable platform for the overall DDSs. While other drivers may excel in specific areas, the actuator combines multiple desirable features, such as precision control, reusability, scalability, low energy consumption and integration with connectivity technologies. These advantages make it a promising solution to address the evolving trends and requirements in SC biologics delivery.
Addressing Unmet Needs and Unique Applications That Existing Traditional Driving Mechanisms Cannot Handle
The actuator’s non-traditional (non-linear) actuation methods, such as gas micropump systems, offer unique advantages for specific applications. By deviating from traditional primary containers like PFSs or cartridges, the actuator opens up possibilities for innovative drug delivery approaches that require different non-linear mechanisms.
Some unique applications are listed below:
- The actuator can meet the size and power requirements of the tiny “smart pill” for oral administration of biologics applications.
- It can also provide a unique non-linear actuation mechanism for a space-efficient wearable/OBI that can deliver ~50 mL, high-viscosity biologics with reusability.
- Lastly, the gas micropump-based actuator can handle microdosing (such as intravitreal injection) applications that require μL scale volume with precision delivery control.
CONCLUSION
In summary, the development of a reusable and recyclable novel MEMS-enabled actuator addresses the emerging trends and unmet needs in SC delivery of biologics. It offers gentle, consistent and powerful performance based on simple electrochemical principles and efficient energy transfer. It allows for the precise control of flow rate and dose, while supporting both linear and nonlinear actuation for traditional and unique applications. The design is flexible and scalable, accommodating a wide range of dose volumes and viscosities. The reusable actuator also includes connectivity and digitisation features that enhance its versatility and sustainability to ensure its readiness for the eco-friendly environment and the upcoming artificial intelligence era.
Driver (Applications) |
Spring (AI OBI IP) |
Motor (AI OBI IP) |
Gas cylinder (AI) |
Elastomeric (OBI, IP) |
MEMS Actuator (AI OBI IP) |
Driving Mechanism | Spring force | Electro/mechanical Motor toque | Pneumatic | Elastic force | Electrochemical Pneumatic |
Energy source | Preloaded spring induced stress |
Battery | Pressurised gas Risks: leakage and high pressure gas storage |
Energy transfer from user to elastic material |
Battery |
Container | PFS, Cartridge | PFS, Cartridge, custom built | PFS | Custom built | PFS, Cartridge, custom built |
Flow rate control | No | Adjustable | Limited | No | Adjustable |
Size | Small to med Size depends on force requirement |
Large When large force is required Space for gear transmission |
Medium | Small | Small MEMS miniaturised |
Cost | Low | High | Med | Low | Med |
Hardware/software | No | High | No | No | Low to med |
Moving parts | Low | High | Med | Low | Low |
Connectivity | Extra | Easy | Extra | Extra | Easy |
Reusability | No | Yes | No | No | Yes |
Platform adaption | Low | High | Low to med | Med | High |
Adaptable to all existing AI, OBI, infusion pump | Low to med | High | Low to med | Low to med | High |
Overall comments | Cheap Simple assemblyHigh impact glass damage Uneven flow rate Large size spring – larger container Single use |
Precision control Flexible platform adaption Connectivity HMI + display ReusabilityExpensive Complex assembly Complex HW/SW Many moving parts Large size & power for large torque Noise due to high revolution |
Soft impact Simple assemblyLong term stored pressured gas Leak risk Gas exhaust management Gas sensitive to environment Limited suppliers |
Cheap Simple assembly Small sizeInconsistent flow rate Limited elastic force strength Single use |
Soft impact Simple assembly Precision control Flexible platform adaption Connectivity HMI + display Low energy Reusability Scalable small to big Recyclable actuator- refillable electrolyteNovel technology – development time |
Table 2: Comparison of the current driving technologies for the SC DDS (autoinjector, OBI, infusion pump).
Performance rating – green: pros, red: cons, blue: mediocre.
REFERENCES
- “Biologics Market Size, Share & Trends Analysis Report By Source (Microbial, Mammalian), By Product (MABs, Recombinant Proteins, Antisense & RNAi), By Disease Category, By Manufacturing, By Region, And Segment Forecasts, 2023 – 2030”. Grand View Research, accessed Jan 2024.
- Lu RM et al, “Development of therapeutic antibodies for the treatment of diseases”. J Biomed Sci, 2020, Vol 27(1), p 1.
- Thomas F, “Rising to the challenge of biologic drug formulation”. Pharm Technol Eur, 2019, Vol 31, pp 24–26.
- “Injectable Drugs Market Outlook (2022 to 2032)”. Future Market Insights, Jun 2022.
- Viola M et al, “Subcutaneous delivery of monoclonal antibodies: How do we get there?”. J Control Release, 2018, Vol 286, pp 301–314.
- Frost GI, “Recombinant human hyaluronidase (rHuPH20): an enabling platform for subcutaneous drug and fluid administration”. Expert Opin Drug Deliv, 2007, Vol 4, pp 427–440.
- Bookbinder LH et al, “A recombinant human enzyme for enhanced interstitial transport of therapeutics”. J Control Release, 2006, Vol 114, pp 230–241.
- Li B et al, “New Engine for Primary Container Injectors: a Powerful Micro Linear Actuator”. ONdrugDelivery, Issue 117 (Mar 2021), pp 82–85.
- Lai JR, Cheng TC, Li B, “A New MEMS Engine for Large-Volume Subcutaneous Injectors”. ONdrugDelivery Issue 137 (Sep 2022), pp 36–41.
- Pivot X et al. “Patients’ preferences for subcutaneous trastuzumab versus conventional intravenous infusion for the adjuvant treatment of HER2-positive early breast cancer: fnal analysis of 488 patients in the international, randomized, two-cohort PrefHer study”. Ann Oncol, 2014, Vol 25, pp 1979–87.
- Bittner B et al, “Subcutaneous administration of Biotherapeutics: An overview of current challenges and opportunities”. BioDrugs, 2018, Vol 32, pp 425–440.
- Henricks LM et al, “The use of combinations of monoclonal antibodies in clinical oncology”. Cancer Treat Rev, 2015, Vol 41, pp 859–67.
- Indrajit G et al, “A systematic review of commercial high concentration antibody drug products approved in the US: formulation composition, dosage form design and primary packaging considerations”. MAbs, 2023, Vol 15(1), article 2205540.
- “Halozyme Announces Positive Clinical Data of its High-Volume Auto-Injector Demonstrating Successful Rapid Subcutaneous Drug Delivery”. Press Release, Halozyme Therapeutics, Oct 16, 2023.
- Schneider A et al., “Hold the device against the skin: the impact of injection duration on user’s force for handheld autoinjectors”. Expert Opin Drug Deliv, 2020, Vol 17(2), pp 225–236.
- “IDF Guide to Ig Therapy”. Immune Deficiency Foundation, Jul 11, 2018.
- Bienvenu B et al, “Rapid Push vs Pump-Infused Subcutaneous Immunoglobulin Treatment: a Randomized Crossover Study of Quality of Life in Primary Immunodeficiency Patients”. J Clin Immunol, 2018, Vol 38(4), pp 503–512.