Citation: Clayton J, Bone P, Mok Hwang K, “Analytical Techniques for the Formulation of Gastroretentive Tablets”. ONdrugDelivery, Issue 122 (Jul 2021), pp 22–27.
Jamie Clayton, Phil Bone and Kyu Mok Hwang discuss the value of analyses such as mercury porosimetry and powder rheology in characterising excipients for gastroretentive tablet formulation, and go on to detail a case study investigating polymer excipients for a novel bilayer floating tablet.
“Prime targets for GR OSD forms are drugs for local action in the stomach, with a NAW or with poor solubility or stability under alkaline conditions. Improving the gastroretention of such drugs can boost efficacy, and at the same time enable a streamlined dosing regime via sustained or controlled release.”
For certain drugs, formulation into a gastroretentive (GR) oral solid dosage (OSD) form can be a successful strategy for improving bioavailability and therapeutic efficacy. Such drugs include those in Biopharmaceutics Classification System (BCS) Class IV, which covers actives with low solubility and low permeability, and as such present some of the toughest formulation challenges. Prime targets for GR OSD forms are drugs for local action in the stomach, with a narrow absorption window (NAW) or with poor solubility or stability under alkaline conditions. Improving the gastroretention of such drugs can boost efficacy, and at the same time enable a streamlined dosing regime via sustained or controlled release. Expandable, swelling, floating, muco-adhesive and high-density systems are all routinely deployed depending on the properties of the drug and the required delivery profile.1
This article considers the specific challenge of developing floating GR OSD forms and the analytical techniques that can be deployed to support their formulation. Experimental work by researchers at the School of Pharmacy, Sungkyunkwan University2 highlights the value and relevance of porosity and powder flowability measurements in the development of novel, bilayer GR tablets.
FORMULATING TABLETS THAT FLOAT IN THE STOMACH
In both the fed and fasting states, the stomach empties on a regular basis, which can be problematic when it comes to the efficacy of OSD forms. Short periods of intense, regular contractions sweep undigested food from the stomach to the small intestine, potentially removing drugs from their preferred location of activity. For drugs that have a NAW in the first part of the gastrointestinal tract (GIT), that are prescribed for local action or that only dissolve or absorb effectively under acidic conditions, this action effectively brings drug delivery to a halt, limiting bioavailability.3
A range of different formulation strategies have been deployed to increase gastroretention, notably for sustained release products. Sustained release formulations are associated with high patient compliance and provide lower, more consistent drug concentrations, reducing the risk of undesirable systemic effects. Technologies that enhance gastroretention increase the viability of sustained release, with floating tablets being the most practical and widely studied approach.1 Formulated to have lower density than the gastric juices (density of approximately 1.0 g/cc), such tablets rise to the top of the stomach to reduce the risk of removal. Examples of commercial floating tablets can be classified on the basis of whether they deploy effervescent or non-effervescent systems, and include products such as Cifran OD® (Ranbaxy, India), an antibiotic, and Madopar NBS® and Prolopa HBS® (both Roche, UK), which are both prescribed for Parkinson’s disease.
The successful formulation of floating GR products relies on controlling the properties of the tablet to achieve the required retention time and drug release profile in vivo, under the specified conditions of use. Tablet size, shape and density can all be optimised to meet these goals, with polymers frequently incorporated to impart buoyancy via swelling or the incorporation of air. However, formulation is complicated by the need to:
- Achieve immediate buoyancy – delayed buoyancy, such as that imparted by slowly swelling polymers, risks premature product loss
- Ensure that the tablet is sufficiently mechanically robust to withstand gastric action for the duration of drug delivery
- Simultaneously control both the drug release profile and tablet residence time – often parameters that are manipulated to improve strength or buoyancy simultaneously impact drug release.
Measurement of the physicochemical properties of excipients and the finished product provides a foundation for the development of a successful product.
WHICH ANALYTICAL TECHNIQUES CAN HELP?
While the formulation of floating GR OSD products has much in common with that of conventional tablets, the need to understand and control buoyancy is distinct. Furthermore, excipients, such as sublimating agents, that can be used to impart porosity (and by extension buoyancy) can increase the cohesiveness of tableting blends, making them harder to process.2 Against this backdrop, the following analytical techniques are noteworthy in terms of their ability to provide valuable insight.
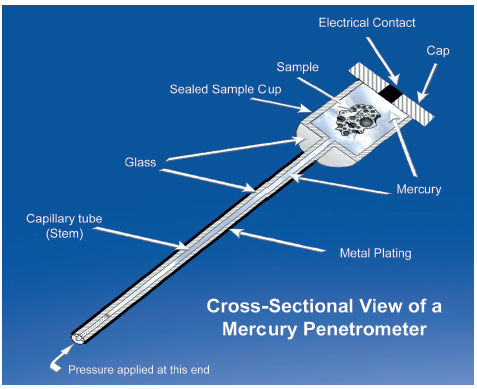
Figure 1: Mercury porosimetry involves measurement of the amount of mercury forced into the pores of a sample as a function of pressure.
Mercury Porosimetry – Detailed, Comprehensive Pore Size Characterisation
Mercury porosimetry quantifies median pore size, pore size distribution and other porosity parameters, from measurements of the amount of mercury that penetrates a sample as a function of pressure (Figure 1). Washburn’s equation describes the direct correlation between pore size and the pressure required for pore filling. Relative to other techniques for porosity characterisation, mercury porosimetry is fast and accurate, offers a very wide dynamic range – from a pore size of 3 nm to 0.36 mm – and generates more detailed results. For example, it can be used to determine tortuosity within a tablet and information about pore shape.
Using mercury porosimetry on tablets generates data that can inform on mechanical strength and fluid transfer within the tablet. Pore structure governs fluid transfer and quantifies the resistance to fluid penetration of the tablet, elucidating its wetting characteristics.4 If a tablet exhibits any elastic behaviour due to the incorporation of polymeric additives, then this would also be observable in a comparison of mercury intrusion and extrusion profiles. Such data can elucidate disintegration behaviour and provide fundamental insights into dissolution performance. For GR OSDs, detailed porosity measurements can make it easier to understand how to simultaneously engineer tablet robustness and buoyancy.
Dynamic Powder Testing – Sensitive, Relevant Powder Flowability Measurement
Powder flowability can be assessed using a range of techniques, including those specified in United States Pharmacopeia (USP) 1174 – shear cell testing, flow through an orifice, angle of repose and tapped density methods. However, experience underlines the superior sensitivity and relevance of dynamic powder testing for many pharmaceutical applications, including tableting.5 Dynamic testing involves the generation of flow energy values from measurements of the axial and rotational forces acting on a precision-engineered blade as it rotates through a powder sample. Powders can be tested in a consolidated, moderate stress, aerated or fluidised state to simulate the process of interest, a capability which sets dynamic testing apart from other methods (Figure 2).
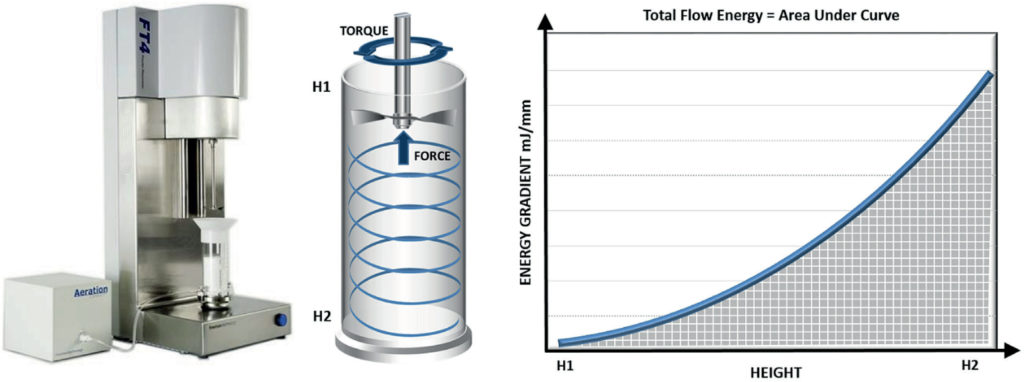
Figure 2: Dynamic testing can quantify the flow behaviour of powders in a consolidated, moderate stress, aerated or fluidised state.
Dynamic properties are measured using well-defined, largely automated protocols, including basic flowability energy (BFE) and specific energy (SE), which respectively quantify the confined (forced flow) and unconfined (gravity flow) flow properties of a powder in a low stress state. Dynamic measurements are highly repeatable and reproducible, and the technique is associated with exemplary sensitivity, with the ability to differentiate powders that other test methods classify as identical.
“Sustained release formulations are associated with
high patient compliance and provide lower, more consistent drug concentrations, reducing the risk of undesirable systemic effects. Technologies that enhance gastroretention increase the viability of sustained release, with floating tablets being the most practical and widely studied approach.”
Flowability is a critical characteristic for tableting blends that impacts the efficiency of die filling and, more generally, the ease and consistency of powder movement through a tableting press. Indeed, flow additives are routinely incorporated into tableting blends to enhance flowability and facilitate the production of high-quality tablets of uniform weight at an economically attractive rate. With dynamic testing it is possible to quantify flow behaviour under different conditions to directly simulate specific steps of the tableting process. Furthermore, since instrumentation for dynamic testing also enables shear and bulk property measurement, it is also possible to generate highly relevant data for hopper discharge (shear cell and permeability) and tablet compression (bulk density, permeability and compressibility).
CASE STUDY: DEVELOPING A BILAYER GR TABLET WITH HYDROPHOBIC POLYMERS
A recent study by researchers at Sungkyunkwan University illustrates the challenges of GR formulation and the insights provided by the aforementioned analytical techniques.2 The goal of the study was to develop a strong bilayer floating GR tablet for the sustained release of rebamipide (RBM). A BCS IV drug used to treat gastric ulcers and gastritis, RBM has low oral bioavailability, due to poor, regionally variable permeation. A GR sustained release formulation has the potential to significantly enhance the therapeutic potential of RBM, which currently relies on high-frequency dosing. In contrast to conventional practice, the researchers chose to investigate the use of a hydrophobic, rather than hydrophilic, polymer to create a strong, inert matrix capable of withstanding gastric action, post-hydration.
Tablets were prepared using two discrete powder blends, one to form the GR layer, the other to form the drug release layer. The GR layer was a blend of polymer – either Kollidon® SR (BASF, Ludwigshafen, Germany) or polyethylene oxide (PEO) 7M (Polyox® WSR 303 – DuPont, Wilmington, DE, US) – and varying levels of camphor (Junsei Chemical, Tokyo, Japan), a sublimating agent. The drug layer was a wet granulated blend of RBM (Estech Pharma, Seoul, South Korea) and dicalcium phosphate (DCP) (Calipharm A®, Innophos, NJ, US) mixed with colorant and polymers to control erosion and the rate of drug release. MgSt (Daejung Chemical, Siheung, Korea) and Aerosil® R972 (Evonik, Essen, Germany) were added to both blends ahead of compaction to improve flowability and tableting performance.
Complete tablets were produced using a two-step process. The first step involved manual filling of the die with the GR layer, followed by pre-compression. The drug layer was then added, and the entire tablet was subject to a compression force in the range 0.5–1.5 tons. Tablets were then dried at 60oC for 12 hours in a vacuum oven to ensure complete sublimation of the camphor prior to stabilisation and subsequent analysis. Single GR layer tablets were also produced for further analysis.
A range of analytical tests was applied to rationalise and optimise the performance of the tablets, including:
- Gas pycnometry (AccuPyc 1340 – Micromeritics) to determine the volume fraction of the polymeric drug release agent in the drug layer
- Scanning electron microscopy (SEM) (JSM-6010 plus LA model scanning microscope – Jeol, Tokyo, Japan) to investigate particle morphology
- Tests to assess tablet performance – hardness, hydrated drug layer strength, buoyancy and dissolution performance.
Porosity Measurements
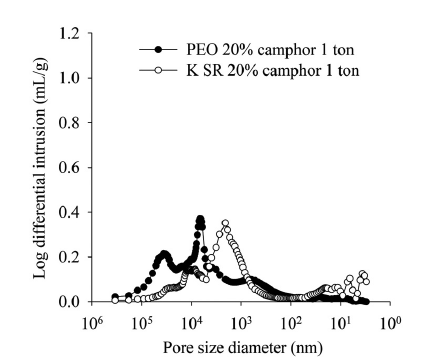
Figure 3: Fluid intrusion for two tablets with differing pore diameters.
Mercury porosimetry measurements (AutoPore IV 9500 V1.09 – Micromeritics) were carried out in accordance with the standard protocols for the instrument to investigate the structure of the GR layer.
The data shown in Table 1 and Figure 3 show the impact of polymer choice on developed porosity. Although average porosity and density are similar in each case, the Kollidon® SR formulation has much smaller pores. This difference may be attributable to the differing morphology of the two polymers; the Kollidon® SR particles are finer – a volume-weighted average particle diameter of around 60 μm compared with 157 μm for the PEO 7M – with a more regular, spherical shape and smoother particle surface (SEM data – not shown). The hollow structure of the Kollidon® SR and small cavities in the particles might also be a contributing factor. In contrast, the PEO 7M particles are larger, denser and more irregular in shape.
Kollidon® SR | PEO 7M | |
Median Pore Diameter (V) (nm) | 1981.5 | 6888.8 |
Median Pore Diameter (Area) (nm) | 4.1 | 12.7 |
Average Pore Diameter (4V/A) (nm) | 40.7 | 295.9 |
Bulk Density (g/mL) | 0.7852 | 0.7653 |
Apparent (Skeletal) Density (g/mL) | 1.1388 | 1.1215 |
Porosity (%) | 31.0491 | 31.7625 |
Table 1: Porosity metrics and pore size distribution data for GR single layer tablets produced using 20% camphor and a 1 ton compression force.
Figure 4 shows data illustrating the impact of camphor concentration and compression pressure on the porosity of Kollidon® SR samples. These results show that total porosity can be increased either by reducing compression force or increasing camphor concentration. The only two formulations that produced floating bilayer tablets were those with the highest total porosity, those produced with 20% camphor at 0.75 tons and 0% camphor at 0.5 tons. The data also suggest that the inclusion of camphor results in an appreciable population of relatively large pores, relative to those produced by the compaction process. These results are again consistent with the SEM data, which show that, after sublimation, the Kollidon® SR matrix, though quite coherent, includes many pores that are substantially bigger than neighbouring particles.
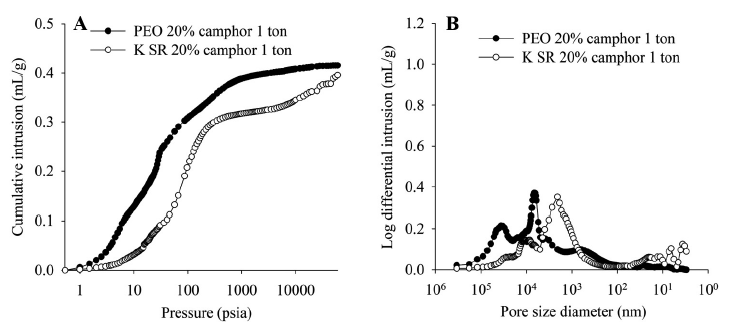
Figure 4: Porosimetry data showing the impact of camphor concentration and compression force.
These results illustrate the importance of measuring pore size distribution rather than averaged porosity metrics, which failed to detect differences between the two polymers. They also show how camphor and compression force can be manipulated to achieve highly porous tablets that also have the strength and hardness required; tablets produced with excessive levels of camphor or at low compression were shown to lack viable hardness. Such insights are critical when developing tablets that combine the required mechanical characteristics with in vivo buoyancy.
Flowability
The flow behaviour of GR blends incorporating optimised levels of camphor was characterised using traditional techniques (angle of repose and Carr’s index) and by dynamic testing (FT4 Powder Rheometer® – Freeman Technology) using standard testing protocols for the instrument (Table 2).6 The dynamic parameters measured include BFE, SE and flow rate index (FRI), which is determined by measuring BFE as a function of blade-tip speed (FRI is defined as BFE at 10 mm/s divided by BFE at 100 mm/s).
Parameters | Kollidon® SR | PEO 7M | |
Conventional Methods |
Angle of Repose (o) | 29 ±1 | 33 ±2 |
Carr’s Index (%) | 8 ±1 | 12 ±3 | |
FT4 Powder Rheometer |
Basic Flowability Energy (mJ) | 55.30 ±0.89 | 295.37 ± 2.21 |
Specific Energy (mJ) | 2.33 ±0.14 | 8.40 ±0.18 | |
Flow Rate Index | 1.53 ±0.04 | 2.75 ±0.47 | |
Conditioned Bulk Density (g/mL) | 0.487 ±0.006 | 0.406 ±0.002 |
Table 2: Flow property data for GR blends incorporating 20% camphor.
Both traditional powder testing methods classify the Kollidon® SR as having ‘excellent’ flow properties compared with the ‘good’ classification of the PEO 7M. However, the variability of these data is such that the two powders are barely differentiated, particularly by Carr’s Index. Furthermore, the results are contrary to expectations on the basis of particle size, since finer particles typically exhibit relatively poor flowability. In contrast, the dynamic and bulk property values exhibit greater repeatability and more robust differentiation. In addition, since dynamic testing enables the simulation of process conditions, these properties can be used to investigate which formulations are likely to perform well in the various steps of a tableting process.
“The development of GR tablets, and more specifically floating tablets, is a promising strategy for active ingredients that are not easily delivered via more conventional OSD forms.”
BFE data reflect flow behaviour under forced conditions, such as in the feed frame of a tablet press. BFE values for PEO 7M are substantially higher than for Kollidon® SR, indicating that it presents greater resistance to flow under these conditions and is more likely to perform poorly in this type of unit operation. This result may be attributed to the smooth, regular morphology of the Kollidon® SR particles, which is typically more conducive to good flow characteristics.
SE data are more relevant to gravitational powder flow, but similarly highlight the greater flowability of the Kollidon® SR. SE values are strongly influenced by interparticle friction and mechanical interlocking. These results again suggest that the PEO 7M is the more cohesive powder and further underline the impact of the morphology of the Kollidon® SR, which is likely to reduce mechanical and frictional forces within the powder.
FRI data similarly indicate the greater cohesiveness of the PEO 7M, since more cohesive powders are generally more sensitive to changes in flow rate because of their greater tendency to entrain and trap air. Bulk density data for the two polymers supports the hypothesis that the Kollidon® SR is more efficiently packed and contains less air. From a practical perspective, these data suggest that the PEO 7M is likely to be more sensitive to changes in processing rate.
In summary, the flow data provide consistent evidence that the Kollidon® SR blend is likely to perform better at every stage of the tableting process, compared with an equivalent PEO 7M blend, with dynamic testing providing more sensitive and relevant insight than can be accessed via traditional methods.
CONCLUSION
The development of GR tablets, and more specifically floating tablets, is a promising strategy for active ingredients that are not easily delivered via more conventional OSD forms. However, formulating such tablets is a demanding task, complicated by the need to engineer both buoyancy and the mechanical strength required to withstand gastric action for an appreciable residence time.
The study presented here shows how mercury porosimetry and powder rheology can be applied to generate data to support excipient choice and successful product development. Both techniques played a role in helping researchers to develop a successful bilayer tablet with a highly porous, hydrophobic GR layer with superior mechanical strength and tableting properties, compared with conventional hydrophilic alternatives.
Figures and tables reproduced from reference two with kind permission of the authors.
REFERENCES
- Tripathi J et al, “Current state and future perspectives on gastroretentive drug delivery systems”. Pharmaceutics, 2019, Vol 11(4), p 193.
- Nguyen TT et al, “Development of novel bilayer tablet based on hydrophobic polymers”. Int J Pharm, 2020, Vol 574, Article 118865.
- Ibrahim M et al, “Gastro-retentive oral drug delivery systems: a promising approach for narrow absorption window drugs”. J Adv Biomed & Pharm Sci, 2019, Vol 2, pp 98–110.
- “The definitive guide to physical characterization for solid dosage forms”. White paper, Micromeritics.
- Clayton J, “Reviewing Current Practice in Powder Testing”. Org Process Res Dev, 2015, Vol 19(1), pp 102–109.
- Lindberg NO et al, “Flowability Measurements of Pharmaceutical Powder Mixtures with Poor Flow Using Five Different Techniques”. Drug Dev Ind Pharm, 2004, Vol 30(7), pp 785–791.