To Issue 170
Citation: Brooks C, “Developing Robust Orally Inhaled Products to Achieve Better Patient Outcomes”. ONdrugDelivery, Issue 170 (Apr 2025), pp 58–62.
Clair Brooks of Copley Scientific looks at some of the in-use issues and patient effects that good design of devices for orally inhaled drug products can address within the context of regulatory requirements for the demonstration of robustness.
It is sobering to imagine the transformation in patient outcomes that could be achieved through the better use of existing therapies for respiratory diseases. Reported non-adherence in asthma sufferers runs at 30–70%,1 with complementary studies of inhaler technique indicating that as many as 50–80% of those suffering from chronic obstructive pulmonary disease and asthma are unable to use their prescribed orally inhaled products (OIPs) properly.2
The potential impacts of this problem are far reaching. In the UK, for example, asthma accounts for 1,200 deaths, 50,000 hospital admissions and £6 billion in lost productivity per year;1 the overuse of reliever medication alone has been associated with an environmental footprint of 250,000 tonnes of CO2 equivalent per year.3 And this problem is a persistent one, with little improvement observed over the last four decades.
Patient non-adherence and product misuse is a complex, multifactorial issue, but device design and performance have a defining role to play in addressing it. Compared with alternative solutions, such as enhanced healthcare practice and digitisation, inherently robust OIPs can be both highly effective and cost-efficient.
THE PERSISTENT PROBLEM OF INHALER MISUSE
The combination product classification applied to OIPs highlights the interplay between device and formulation that defines performance. However, in practice, there is a third factor in play – the patient. When it comes to clinical efficacy, it is the interplay between device, formulation and patient that defines the dose received and its therapeutic effect. This sets inhaled drug delivery apart from other administration routes and is an important area of focus for OIP developers.
Patient-related effects may be linked to patient physiology and disease state, or to patient behaviour – intentional or otherwise. For example, the breathing profile applied during product use is a function of the patient’s inherent breathing capabilities (physiology and disease state) and how adept they are at performing a specified inhalation manoeuvre (behaviour).
“IT IS TEMPTING TO BELIEVE THAT PATIENTS CAN BE ‘TRAINED OUT’ OF BEHAVIOURAL IMPACTS, BUT EXPERIENCE AND STATISTICS SUGGEST OTHERWISE.”
It is tempting to believe that patients can be “trained out” of behavioural impacts, but experience and statistics suggest otherwise. Studies show that many healthcare professionals are unable to demonstrate correct inhaler technique2 and time constraints add to the difficulty of delivering sufficient, effective training and refresher training. Despite efforts to improve and the high degree of detail required in information for use instructions, device misuse remains stubbornly high.
One explanation for this is that some misuse is purposeful. A patient that fails to recognise the importance of preventer medication may be poorly motivated to adhere rigorously to a regime that shows little outward sign of effect. Cost may also be a factor in some geographies, leading to the intentional “preserving” of medication. On the other hand, there are undoubtedly patients that are trying hard to use their OIPs as directed and failing.
Better device design has a role to play in reducing this problem. Connected and digital devices are one approach that has a growing track record of success. For example, trials of Hailie® (Adherium, Melbourne, Australia) with child patients show that it can reduce both the use of rescue medication and hospital admissions by 45% and 80%, respectively, and a UK NHS study to improve outcomes for high-risk children and young adults is currently underway.4–6 Simply by recording usage patterns and prompting adherence, such solutions can make a major difference to patient outcomes, with the ability to monitor applied inhalation profiles an additional important benefit for dry powder inhalers (DPIs). That said, cost is a major limitation with respect to uptake, depending on healthcare structure and funding.
Designing more robust performance into conventional inhalers is arguably the more broadly beneficial alternative. In the first instance, doing so relies on identifying common issues associated with patient behaviour and inhaler use, and developing testing strategies to investigate them.
THE REGULATORY PERSPECTIVE
US FDA and EMA guidance includes a range of drug characterisation studies that reflect the potential for variability associated with patient physiology, behaviour and day-to-day use.7–9 Depending on the specific OIP and guidance being followed, examples include:
- Shaking requirements for suspension-based formulations, notably metered dose inhalers (MDIs)
- Initial priming and re-priming requirements, following storage in various orientations
- Low-temperature performance, following storage at sub-zero temperatures
- Performance after temperature cycling, between sub-zero and above room temperature
- Effect of environmental moisture.
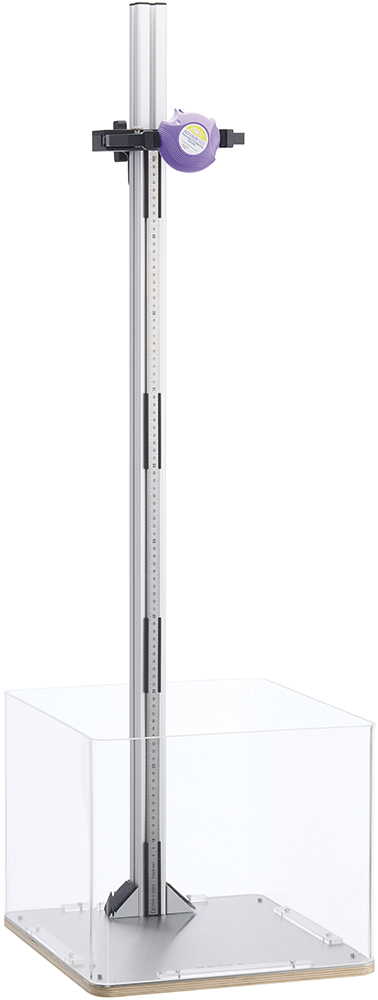
Figure 1: Copley’s Drop Test Apparatus provides a repeatable and reproducible solution for drop-testing in accordance with the guidance provided in ISO 20072.
The need to assess robustness is also specifically listed with associated studies designed to confirm “that the MDI or DPI product is of sufficiently robust design to withstand shipping conditions and typical patient usage”.7 The additional information supplied to support study design references the need to assess the impact of actions such as dropping, agitation and shipping, with the latest draft of the EMA guidance providing the most detail in this area.9 It highlights the value of:
- Assessing the performance of the device when activated at the frequency indicated in the product information
- Demonstrating the robust performance of any lock-out device or digital sensor
- Determining the vibrational stability of powder mixtures
- Carrying out dropping simulation studies with products that are towards their end of life (e.g. at dose 180 for a 200-dose product), due to the potential impact of releasing the accumulated drug (Figure 1).
The extent to which these tests accurately capture the impact of “typical patient usage” defines their value for those working to make devices as robust as possible, who may also choose to investigate beyond the indicated studies. Focusing on specific behavioural issues widely known to cause variability in drug delivery can deliver additional insights to support innovation towards more robust performance in the broadest sense of the word.
“FOCUSING ON SPECIFIC BEHAVIOURAL ISSUES WIDELY KNOWN TO CAUSE VARIABILITY IN DRUG DELIVERY CAN DELIVER ADDITIONAL INSIGHTS TO SUPPORT INNOVATION TOWARDS MORE ROBUST PERFORMANCE IN THE BROADEST SENSE OF THE WORD.”
STUDY SPOTLIGHT: INVESTIGATING THE EFFECT OF EXHALATION THROUGH A DPI
The passive nature of DPI devices makes them uniquely sensitive to the inhalation manoeuvre applied during use. This is reflected in:
- Compendial methods for DPIs, for which flow rate is determined from the resistance of the device, rather than being fixed
- The requirement for product characterisation studies across a range of flow rates that are relevant to the target patient population7 or in the 30–90 L/min range9
- Studies of the impact of the applied flow rate profile, notably the rise time to peak inspiratory flow rate, by product developers adopting more clinically relevant test methods.10
However, it is not just inhalation during product use that can be a source of variability; the exhalation manoeuvre that precedes it can be too. With DPIs, exhaling to functional residual capacity, or just below, empties the lungs, readying them for the deep inhalation required for successful drug delivery. Unfortunately, studies show that many patients prepare incorrectly, with failures at the exhalation stage highlighted as the most common error associated with DPI technique.11–13
“FAILURE TO EXHALE AWAY FROM THE INHALER WAS OBSERVED IN APPROXIMATELY 80% OF PATIENTS IN ONE STUDY, WHILE THE SPECIFIC ISSUE OF EXHALATION THROUGH THE INHALER IS REPORTED IN 14–22% OF UNSUPERVISED PATIENTS.”
Failure to exhale away from the inhaler was observed in approximately 80% of patients in one study,14 while the specific issue of exhalation through the inhaler is reported in 14–22% of unsupervised patients.15 Furthermore, there is evidence of a lack of understanding of the importance of exhalation by healthcare professionals and considerable resistance to improvement by training.15
This is problematic given the potential for exhalation into the device to blow out the dose and, more critically, the susceptibility of DPI formulations to moisture, notably with respect to their tendency to agglomerate. DPI formulations and devices are matched to ensure that a correctly applied inhalation manoeuvre supplies sufficient energy to disperse the formulation to a respirable particle size. If the energy needed to achieve such dispersion increases, due to stronger inter-particulate bonds, then more of the drug will be delivered in the form of larger and/or agglomerated particles and therefore not reach the lung.
Experimental studies support this hypothesis. For example, Holmes et al found that delivered dose fell to less than 50% of the label claim when air with a relative humidity of 80% was introduced into a Diskus™ DPI (salmeterol/fluticasone, GSK) prior to inhalation.15 Measurements of the relative humidity of exhaled air have shown it to vary widely across a range from approximately 40% to 90%, so this figure is clinically representative with respect to studying the impact of an incorrect exhalation manoeuvre.16
In this study, the exhalation profile applied to the device was varied with respect to:
- Flow rate (30, 60, 90 and 120 L/min)
- Distance between mouthpiece and air source (0, 5 and 10 cm)
- Duration (2, 4 and 6 s)
- Relative humidity (RH) of the air used (28% and 80%).
Delivered dose testing was carried out using standard compendial methods to assess the impact of all four variables on drug delivery, relative to the label claim.
The results showed that blowing air into the device ahead of use has a negative impact in all scenarios but is especially problematic when the air has a high RH. The introduction of humid (RH 80%) as opposed to dry (RH 28%) air was found to make drug delivery more variable and unpredictable, and to reduce the average amount of drug delivered. Distance from the inhaler was the single most influential factor, with less than 50% of label claim delivered on average following the introduction of humid air at a distance of 0 cm, at all flow rates. At a distance of 5 cm, a similar deterioration in drug delivery was observed at flow rates above 30 L/min.
“THE MAGNITUDE OF THE POTENTIAL IMPACT OF THIS COMMON ERROR IN DPI USE THEREFORE HIGHLIGHTS THE EXTENT TO WHICH EFFECTS BEYOND THOSE ROUTINELY STUDIED UNDER THE HEADING ‘TYPICAL PATIENT USAGE’
CAN COMPROMISE DRUG DELIVERY.”
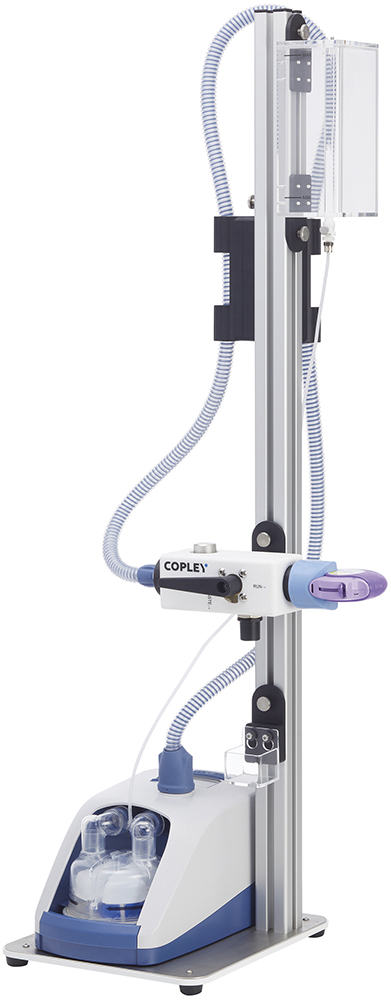
Figure 2: Copley’s Patient Exhalation Simulator for assessing the impact of exhalation on DPI performance to support the development of products with improved robustness and/or demonstrated equivalence.
To investigate this effect in more detail, back-to-back aerodynamic particle size distribution (APSD) measurements were recorded. Here, introducing humid air at a flow rate of 60 L/min for a duration of 4 s at a distance of 5 cm was shown to decrease fine particle fraction (FPF), the >5 μm fraction of the dose, by approximately 40% relative to results obtained in the absence of an exhalation step. This is attributable to clumping or agglomeration of the powder formulation and an associated increase in particle size.
FPF is a primary focus for OIP developers because of the tendency for particles in this size fraction to deposit in the lung. A low FPF is associated with reduced deposition in the lung and a corresponding decrease in clinical efficacy or a higher likelihood of over-prescribing and an increased risk of side effects. The magnitude of the potential impact of this common error in DPI use therefore highlights the extent to which effects beyond those routinely studied under the heading “typical patient usage” can compromise drug delivery. Analytical tools, such as Copley’s Patient Exhalation Simulator (Figure 2), are correspondingly valuable for those working to eliminate potential consequences of misuse.
TOWARDS PATIENT INDEPENDENT PERFORMANCE
Developing a better understanding of how OIPs and, similarly, nasal drug products work for different individuals applying different techniques is becoming a common theme across the inhalation community. FDA product-specific guidelines now routinely invite the use of small and large throats and representative breathing profiles to measure realistic APSDs and confirm bioequivalence in OIPs across a range of patient groups. Additionally, scoping the impact of device orientation is proving critical for those targeting regional deposition in the nasal cavities.
The focus of such studies is often the detection of variability and difference, but the same methods support efforts to develop products that are less sensitive to patient misuse – to go beyond existing definitions of robustness to ensure that products are designed from the ground up to work consistently for all patients types, even if technique is less than optimal. There is merit in making OIPs and nasal products of all types as robust as possible, and a growing number of testing solutions are being developed to support those looking to do so.
REFERENCES
- “Adherence to medication in patients with Difficult Asthma”. Research Report, UK NHS, 2014.
- Volerman A, Carpenter D, Press V, “What can be done to impact respiratory inhaler misuse: exploring the problem, reasons, and solutions”. Expert Rev Respir Med, 2020, Vol 14(8), pp 791–805.
- “New data show overuse of reliever medication in asthma is responsible for 250,000 tonnes of greenhouse gas emissions every year in the UK”. Press Release, AstraZeneca, Feb 2021.
- Chan AHY et al, “The effect of an electronic monitoring device with audiovisual reminder function on adherence to inhaled corticosteroids and school attendance in children with asthma: a randomised controlled trial”. Lancet Respir Med, 2015, Vol 3(3), pp 210–219.
- Morton RW et al, “STAAR: a randomised controlled trial of electronic adherence monitoring with reminder alarms and feedback to improve clinical outcomes for children with asthma”. Thorax, 2017, Vol 72(4), pp 347–354.
- “UK First as new NHS study of digital ‘smart inhalers’ for children with asthma starts in Leicester”. Press Release, NIHR, Jul 2024.
- “Guidance for Industry: Metered Dose Inhaler (MDI) and Dry Powder Inhaler (DPI) Drug Products–Quality Considerations”. US FDA, 2018.
- “Pharmaceutical quality of inhalation and nasal products – Scientific guideline”. EMA, 2006.
- “Guideline on the pharmaceutical quality of inhalation and nasal medicinal products (Draft)”. EMA, 2024.
- Daniels G, Hamilton M, “Assessment of early screening methodology using the Next Generation and Fast Screen Impactor systems”. Poster Presentation, DDL, 2011.
- Lavorini F et al, “Effect of incorrect use of dry powder inhalers on management of patients with asthma and COPD”. Respir Med, 2008, Vol 102(4), pp 593–604.
- Westerik JAM et al, “Characteristics of patients making serious inhaler errors with a dry powder inhaler and association with asthma-related events in a primary care setting”. J Asthma, 2016, Vol 53(3), pp 321–329.
- Pessôa CLC et al, “Most frequent errors in inhalation technique of patients with asthma treated at a tertiary care hospital”. Einstein (Sao Paulo), 2019, Vol 17(2), art eAO4397.
- Press VG et al, “Misuse of respiratory inhalers in hospitalized patients with asthma or COPD”. J Gen Intern Med, 2011, Vol 26(6), pp 635–642.
- Holmes MS et al, “An Acoustic-Based Method to Detect and Quantify the Effect of Exhalation into a Dry Powder Inhaler”. J Aerosol Med Pulm Drug Deliv, 2015, Vol 28(4), pp 247–253.
- Mansour E et al, “Measurement of temperature and relative humidity in exhaled breath”. Sensor Actuat B-Chem, 2020, Vol 304, art 127371.