To Issue 143
Citation: Allmendinger A, Mahler H-C, “Intravitreal Injections – More Than Meets the Eye: a Technical View of Product Use”. ONdrugDelivery, Issue 143 (Mar 2023), pp 38–42.
Andrea Allmendinger and Hanns-Christian Mahler highlight the technical challenges of intravitreal administration and considerations from the ophthalmologist’s and patient’s perspectives.
“Prefilled primary packaging options circumvent the need for additional aseptic preparation steps.”
Severe retinal disorders such as diabetic retinopathy and age-related macular degeneration can now be treated with biological drugs, such as anti-vascular endothelial growth factor (anti-VEGF). Such products need to be administered into the vitreous humour in the back of the eye.
In previous articles, we discussed that ophthalmic products require a high level of process knowledge and state-of-the-art manufacturing technologies and expertise to maximise safety, meet stringent regulatory requirements and minimise cost of goods.1 We also highlighted new regulatory and quality requirements for intravitreal (IVT) applications, and reflected on technical challenges during drug product technical development and manufacturing.2
In this article, we will highlight technical challenges associated with IVT product use and administration, and include considerations from both the ophthalmologist’s point-of-view (e.g. selection of needle size, small injection volumes) as well as from the patient’s perspective.
“ten23 health is capable of precisely filling very small volumes for IVT applications at the highest filling accuracy in the absence of an air bubble while minimising product losses.”
SELECTION OF SYRINGE AND NEEDLE
Dosage forms intended for IVT administration are typically supplied in vials or in a ready-to-use prefilled syringe (PFS) configuration made of glass or a cyclic olefin polymer or copolymer (COP or COC, respectively). Prefilled primary packaging options circumvent the need for additional aseptic preparation steps, such as withdrawal of the drug product solution from the primary packaging container (vial), which minimises the risk of contamination, and hence benefits patient safety.
However, in contrast to many other parenterally used syringes, which are typically supplied with a staked-in needle, IVT prefilled dosage forms are usually supplied without an attached needle but with a Luer-lock connection. The reason is that many ophthalmologists want to select their own needle of preference and may choose between 31G (gauge), 30G and, in very rare cases, even 27G or bigger.
From a drug product technical development perspective, a higher gauge number represents a smaller needle inner diameter and leads to higher injection forces compared with a smaller gauge. According to Hagen-Poiseuille’s law, injection forces depend on the inner needle diameter by the power of four. It should be noted that only outer needle diameter is harmonised with the “gauge” terminology, but inner needle diameter is not. Hence, it is likely that needles from different suppliers that are both nominal “30G” needles, behave very differently in terms of injection force and/or injection time and hence usability and administrability.
Besides the needle inner diameter, injection forces during IVT administration are also influenced by syringe diameter, needle length, injection speed and formulation viscosity. The latter should ideally be minimised during formulation development. However, viscosity is also dependent on temperature. This implies that not only should injection forces be studied during development at intended use (room) temperature, but also at intended storage conditions, typically between 2°C and 8°C, in case the drug product has not been equilibrated adequately, in order to ensure adequate injection forces. Maximum and target injection forces need to be determined based on user preference, e.g. in usability studies. However, they should typically not be much higher than 10 N. Importantly, viscosity is not just a value at a given temperature. Protein formulations may have a very variable viscosity and such formulations may exhibit Newtonian or Non-Newtonian flow behaviour, which significantly influences injection forces.3
INJECTION VOLUME
Injection volumes for IVT application typically range between 25 and 50 μL. However, injection volumes as high as 100 μL have been reported, although little is known about the upper limit of injectable volume to the back of the eye.4 The lower limit of injected volume is usually defined by the precision of injection devices, which is currently around 10 μL for conventional syringes used, depending on graduation marks and proficiency of the target users.
From a technical perspective, filling small volumes into primary packaging containers during fill-finish operations is challenging, both from a filling accuracy perspective as well as from a visual inspection point of view (Figure 1).
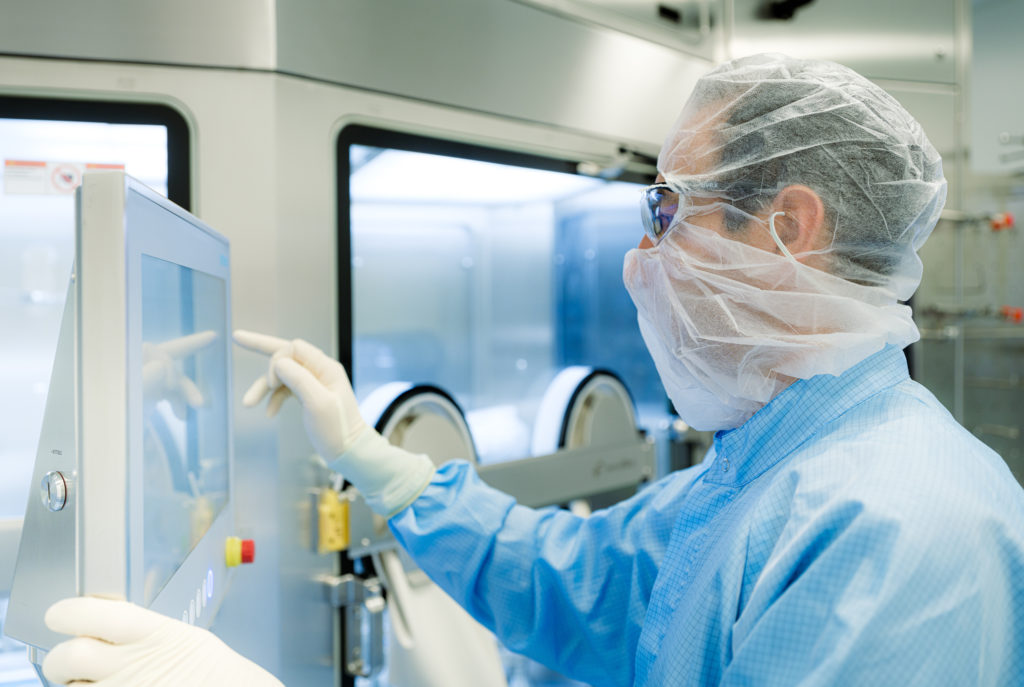
Figure 1: Operator at the filling line in Visp, Switzerland.
IVT dosage forms are, in most cases, overfilled, hence requiring down-dosing to the desired injection volume indicated by a graduation mark according to the instructions for use. As an example, syringes filled with 100 μL target fill volume would, for example, need to be down-dosed to a target injection volume of 50 μL, which, on the one hand, provides a user challenge in terms of precision of the down-dosing, but also generates significant product waste, adversely contributing to overall cost of goods. To ensure dosing accuracy, syringes intended for IVT injection must be appropriately characterised (Figure 2).5,6 In addition, the absence of an air bubble in the syringe is highly desired from a user perspective as down-dosing may be considerably more difficult.
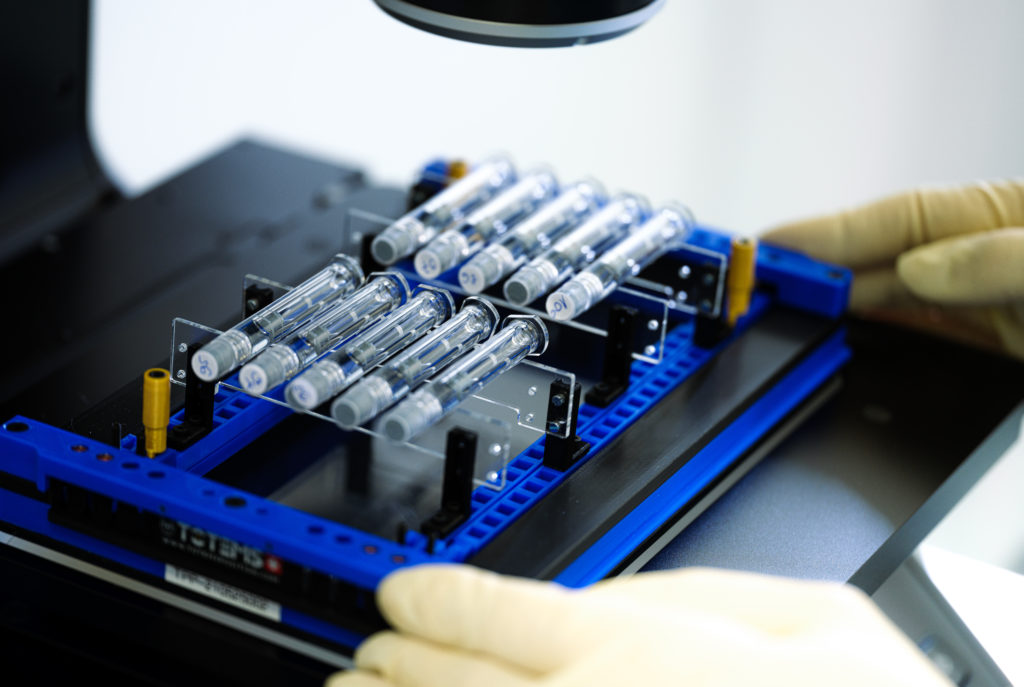
Figure 2: High-accuracy image dimension measurement system, 0.5 mL syringes.
ten23 health is capable of precisely filling very small volumes for IVT applications at the highest filling accuracy in the absence of an air bubble while minimising product losses.
“Formulation excipients, as well as drug product impurities, need to be carefully controlled to avoid exacerbating immune responses or even non-drug-related toxicities.”
PATIENT SAFETY
1. Microbiological Considerations
Injections into the vitreous humour require the highest quality standards to prevent microbiological infections during treatment. Sterility of the drug product is a prerequisite for any parenteral preparation, but considerations for IVT preparations often exceed these standards. Many ophthalmologists ask for a “sterilised syringe” (i.e. sterilisation of the outside of the syringe), which is currently not a formal regulatory requirement. As a result, filled PFS for IVT use are typically decontaminated on the outside in their secondary packaging. This is especially interesting given that many ophthalmologists often do not perform IVT injections in a sterile environment, meaning microbiological contamination after opening of the secondary packaging may occur.
Outside decontamination can be performed by gas sterilisation, for example, by vaporised hydrogen peroxide, ethylene oxide, peracetic acid or nitrogen dioxide. From a technical perspective, gas sterilisation of a filled drug product is challenging as the product quality needs to be maintained. The secondary packaging has to ensure gas permeability.
For pharmaceutical manufacturers, it is important to define target criteria for such outside decontamination processes, including: (a) bioburden before the gas sterilisation process and overall considerations on how to minimise and limit these (low bioburden handling from point of fill to point of secondary packaging); (b) temperature and hold times during processing; (c) target bioburden after the process (is the target to achieve low bioburden or sterility?); and (d) acceptance criteria for any observed quality changes of the product. As an example, polymer syringes, as well as rubber stoppers, are typically highly gas permeable. Hence, it must be assumed that gas may also enter the product solution and may lead to product alterations, such as modifications of the active ingredient, e.g. protein aggregates, adducts or fragments. High-end mass spectrometry is typically required to monitor such product alterations, and typical specification tests may not detect such alterations of the protein. In addition, the gas and process conditions may also lead to alterations of the primary packaging container itself. However, pharmaceutical manufacturers and regulatory authorities should probably carefully assess whether performance of outside decontamination of the syringe in its secondary packaging – which is likely to be contaminated after opening by the user – outweighs any product alteration triggered by the decontamination process.
Besides microbiological product quality, endotoxin levels in the final product are key, especially for IVT preparations. There are many great publications about the effect and no-observed-adverse-effect levels (NOAEL) of endotoxins after IVT injection into preclinical test species.
The NOAEL in rabbits and monkeys has been reported to be as low as 0.01 EU/mL.7,8 These NOAEL are even lower than the levels discussed by the US FDA Guidance for Industry (2015).9 Thus, it is expected that regulatory requirements for endotoxins in IVT preparations are likely to be tightened, given the potential toxicity in the eye. As a result, endotoxin contamination in the API, excipients, as well as primary packaging must be carefully assessed for IVT preparations. Considering that many PFS for IVT injections are “ready to use”, a key focus needs to be on setting acceptable limits for endotoxins (“specifications”) in such container closure systems and on how these relate to a final drug product specification of “NMT (not more than) 0.01 EU/mL”.
2. Excipients
Formulations for IVT applications are developed to best stabilise the active ingredient during manufacturing, storage and transport of the drug product, while ensuring pharmacopoeial and regulatory compliance and physiological tolerance in addition to adequate viscosity. However, the choice of excipients for parenteral preparations is quite limited as they must be tolerated and safe, i.e. non-toxic and non-immunogenic at the intended dose, and approved for parenteral use.
In addition, not all excipients approved for parenteral use are Generally Recognized As Safe (GRAS) for IVT use. For example, methionine acting as a stabiliser or antioxidant in many parenteral formulations has not previously been used for IVT injections, so it should not be used in formulations for IVT administration without very careful and detailed safety assessments. In addition, further consideration should be given to immunogenicity in the eye. Not only excipients, but also drug product impurities, need to be carefully controlled to avoid exacerbating immune responses or even non-drug-related toxicities.10 In addition, excipients and APIs in IVT formulations may also interact with components of the vitreous humour in vivo.11,12
3. Particulate Matter
Particulate matter is regulated for any parenteral preparation. Particulate matter is specifically regulated for IVT preparations, although no controlled preclinical studies have been conducted that relate to the medical relevance of particulates after IVT injection, and there is no other clear clinical evidence. A major concern is if such particulates impact patients’ vision, and particle properties, for example, if floating or if to dissolve in the vitreous humour, need to be considered.13 Requirements for IVT preparations in the European Pharmacopoeia (Ph Eur) are aligned with the requirements of other parenteral preparations (0520). These require products to be “practically free of particles” (Visible Particles, Ph Eur 2.9.20, further discussed in the recommendation chapter 5.17.2.), and the following requirements for sub-visible particulates (Ph Eur 2.9.19) with a maximum of 6000 particles/container for particle sizes ≥10 μm and a maximum of 600 particles/container for particles ≥25 μm. This is significantly different from the US Pharmacopeia (USP), chapter <789>, which suggests significantly tighter limits for IVT preparations, e.g. not more than (NMT) 50 particles mL for particle sizes ≥10 μm and NMT 5 particles/mL for particles ≥25 μm (when using light obscuration testing). This is especially interesting, given that particles may originate from the formulation or primary packaging itself.
Glass syringes typically used for IVT injections are normally siliconised to ensure lubrication and functionality for the user. Hence, unsurprisingly, silicone oil droplets are likely to be detected as (sub-visible and possibly visible) particles in the final drug product after (simulated) injection. The amount of silicone oil used for lubrication, along with the formulation and the storage time of the PFS, may impact the level of silicone detected after (simulated) injection. The measurement of such silicone droplets as particulates is a confounding factor (false positive) during the analysis of particulate matter (Figure 3). Flow imaging analysis and other morphological characterisation tools can easily support the identification of silicone droplets versus any actual particulate contamination.
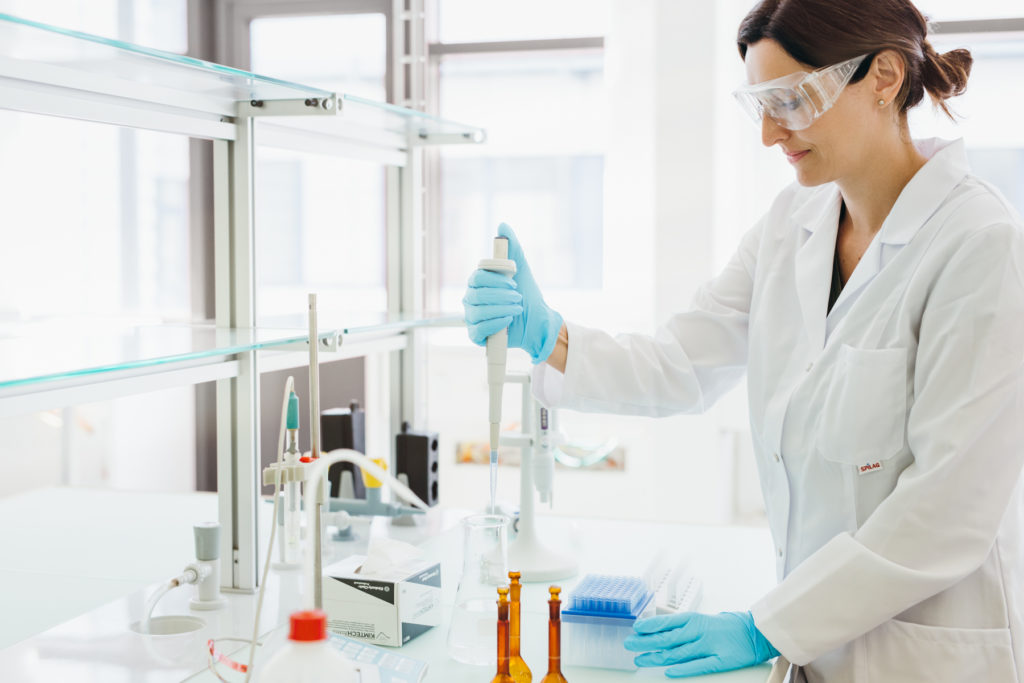
Figure 3: Laboratory in Basel, Switzerland.
“IVT injections bring hope for the treatment of numerous severe retinal disorders, but frequent injections – in some cases weekly or every other week – are also a burden for the patient.”
Given the very low acceptance criteria suggested by USP <789>, many companies try to avoid such analytical findings and hence want to avoid siliconised syringes, especially for IVT use, and evaluate or choose non-siliconised primary containers (syringes).
To note, although occasionally discussed as of concern, minute amounts of silicone droplets are typically not considered as being of any significant medical concern.14 Interestingly, silicone is also used for optical surgery, where approximately 2–3 mL silicone is used in the eye.15
PATIENT CONVENIENCE
Dosing Frequency
From a patient’s perspective, IVT injections bring hope for the treatment of numerous severe retinal disorders, but frequent injections – in some cases weekly or every other week – are also a burden. To increase patient convenience and minimise any risks related to the number of treatments, pharmaceutical manufacturers should be motivated to explore products that offer less frequent injection.
A possible approach relates to sustained releasee, which can be achieved by (a) a modified formulation, (b) alterations to the API (for example by conjugation) or (c) by use of a medical device. From a technical development perspective, sustained release can be highly challenging and requires extensive product characterisation studies to succeed. Very good overviews of sustained-release options and associated challenges are provided elsewhere.16,17
CONCLUSION
IVT preparations present various pharmaceutical and user challenges. ten23 health’s development and aseptic manufacturing services can appropriately and proactively address the needs and complexities associated with IVT and other parenteral preparations.
REFERENCES
- Glöckler L, Delauney C, “State-of-the-Art Solutions for Ophthalmic Sterile Drug Product Manufacturing.” ONdrugDelivery, Issue 118 (Apr 2021), pp 8–10.
- Allmendinger A, Mahler H-C, “How ten23 health is Meeting Developmental Challenges for Intravitreal Products”. ONdrugDelivery, Issue 130 (Mar 2022), pp 43–48.
- Allmendinger A et al, “Rheological characterization and injection forces of concentrated protein formulations: An alternative predictive model for non-Newtonian solutions”. Eur J Pharm Biopharm, 2014, Vol 87(2), pp 318–328.
- Parenky AC, “Container Closure and Delivery Considerations for Intravitreal Drug Administration”. AAPS PharmSciTech, 2021, Vol 22(3), p 100.
- Peláez S et al, “Characterization of Polymeric Syringes Used for Intravitreal Injection”. J Pharm Sci, 2020, Vol 109(9), pp 2812–2818.
- Weinmann C et al, “Role of Formulation Parameters on Intravitreal Dosing Accuracy Using 1 mL Hypodermic Syringes”. Pharm Res, 2020, Vol 37(10), p 190.
- Bantseev V et al, “Determination of a No-Observable Effect Level for Endotoxin Following a Single Intravitreal Administration to Dutch Belted Rabbits”. Invest Ophthalmol Vis Sci, 2017, Vol 58(3), pp 1545–1552.
- Bantseev V et al, “Determination of a No Observable Effect Level for Endotoxin Following a Single Intravitreal Administration to Cynomolgus Monkeys”. J Ocul Pharmacol Ther, 2019, Vol 35(4), pp 245–253.
- “Guidance for Industry and Food and Drug Administration Staff. Endotoxin Testing Recommendations for Single-Use Intraocular Ophthalmic Devices”. US FDA, Aug 2015.
- Wakshull E et al, “Advancements in understanding immunogenicity of biotherapeutics in the intraocular space”. AAPS J, 2017, Vol 19(6), pp 1656–1668.
- Patel S et al, “Evaluation of protein drug stability with vitreous humor in a novel ex-vivo intraocular model”. Eur J Pharm Biopharm, 2015, Vol 95(Pt B), pp 407–417.
- Patel S et al, “Prediction of intraocular antibody drug stability using ex-vivo ocular model”. Eu J Pharm Biopharm, 2017, 112, pp 177–186.
- Patel S et al, “Evaluation of protein drug performance with vitreous humor in a novel ex-vivo intraocular model”. iOVS, 2015, Vol 56(7), p 258.
- Antoun J et al, “Vitreoretinal surgery with silicone oil tamponade in primary uncomplicated rhegmatogenous retinal detachment”. Retina, 2016, Vol 36(10), pp 1906–1912.
- Bakri SJ et al, “Intravitreal silicon oil usage after intravitreal drug injection”. Retina, 2008, Vol 28, pp 996–1001.
- Hughes P et al, “Intraocular delivery considerations of ocular biologic products and key preclinical determinations”. Expert Opin Drug Deliv, 2023, Vol 20(2), pp 223–240.
- Kansteiner F, “Roche recalls new eye therapy Susvimo on leakage fears, aims for market return ‘within a year or so’”. Fierce Pharma, Oct 2022.