To Issue 131
Citation: Brenker J, Alan T, Traini D, “Personalised Aerosol Loading and Management (PALM): a Handheld Device for Aerosol Drug Delivery”. ONdrugDelivery, Issue 131 (Apr 2022), pp 44–48.
Jason Brenker, Tuncay Alan and Daniela Traini introduce the PALM – Personalised Aerosol Loading and Management – device, a unique, advanced drug delivery device enabling personalised delivery of aerosols to improve the efficacy of inhaled medications and patient compliance.
The authors will present a poster about this work at RDD 2022, Orlando, FL, US, May 1–5, 2022, entitled: “Microfluidic Aerosol Generation: Development of a Next-Generation Handheld Device For Aerosol Drug Delivery”.
INTRODUCTION
Respiratory drug delivery is primarily used for the treatment of chronic diseases, such as asthma and chronic obstructive pulmonary disease (COPD), both of which are inflammatory diseases of the lower airways. Over 13% of Australians and 16% of Americans are affected by these diseases and, despite new treatments, they continue to be among the leading causes of deaths in Australia (Australia, 11,202 people in 2019) and internationally (US, 224,987 people in 2019).1
There is also growing interest in using the extensive surface area of the lungs as a route for the systemic delivery of other therapeutic agents. This needle-free alternative to traditional oral formulation is especially advantageous for improving the absorption of poorly bioavailable drugs and reducing side effects. The region of the airway that needs to be targeted depends on the disease to be treated; generally, drugs used to treat inflammatory diseases, such as asthma, should be targeted to the bronchi, the walls of which consist of smooth muscles, whereas drugs for systemic delivery via the lungs should target regions deeper into the alveoli, which are rich in systemic capillaries (Figure 1).
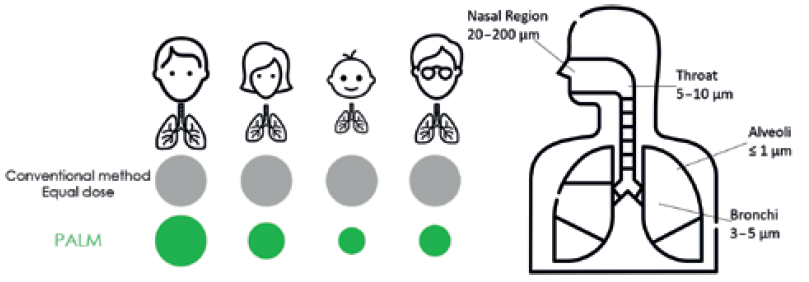
Figure 1: A comparison of the proposed design with existing approaches (left) and an indication of the typical deposition location of different particle sizes in the airways (right).
“The PALM device, with its microfluidic droplet generation and unique patented design, employs a small form-factor breathing device that sits comfortably in the palm and fits a variety of face shapes thanks to its universal design, created specifically to improve user experience and patient monitoring.”
Requirements of Respiratory Drug Delivery and Unmet Needs
The complex, convoluted geometric properties of both the lower and upper respiratory tracts provide numerous distinct challenges for the effective delivery of aerosolised drug compounds. Drug deposition in the respiratory tract primarily depends on three key parameters:
- The size and distribution of the aerosol particles/droplets.
- The rate of delivery of the aerosol to the patient.
- The patient’s breathing pattern.
Particles with aerodynamic diameters in the range of 0.5–5 μm have the highest likelihood to reach the terminal bronchioles and alveolar regions, with larger particles (>5 μm in aerodynamic diameter) likely to become entrapped in the upper airways. However, optimum particle size varies from patient to patient due to differing lung capacities, disease states, age and gender (Figure 1).2 Hence, a personalised approach capable of tuning the particle size is required for increased effectiveness. This is not currently possible with any commercially available device.
An Innovative Particle Delivery Method
To meet this need, researchers from Monash University, Macquarie Medical School and the Woolcock Institute of Medical Research have recently invented the Personalised Aerosol Loading and Management (PALM) device, a portable integrated aerosol system that can address the specific needs of patients for a specific delivery route (Figure 2). The device gives physicians precise control over their patient’s treatment by tuning the particle size and provides users with an easier, more effective treatment.
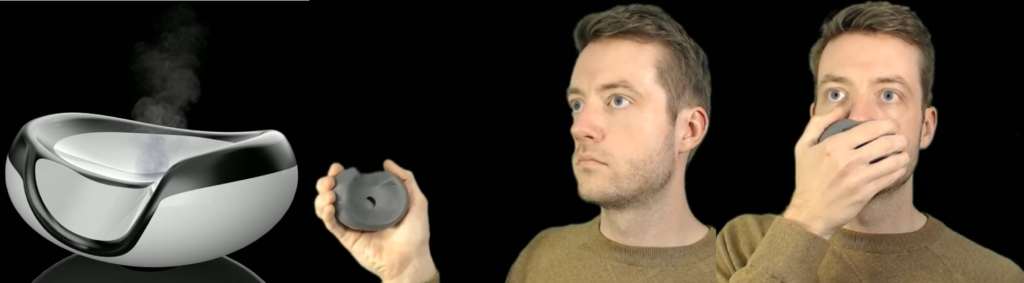
Figure 2: Rendering of the PALM device (left) and image of the PALM device held in the hand (centre) and on the face (right).
The PALM device, with its microfluidic droplet generation and unique patented design, employs a small form-factor breathing device that sits comfortably in the palm and fits a variety of face shapes thanks to its universal design, created specifically to improve user experience and patient monitoring. Using built-in sensors, the PALM device can measure a patient’s breathing cycle and deliver medication at exactly the right moment, eliminating co-ordination issues. Moreover, the particle size can be controlled on-demand to address the specific needs of patients. These capabilities ensure that the PALM device offers the greatest potential for successful lung deposition.
“Current device design has resulted in poor patient compliance and, consequently, poor treatment outcomes.”
BACKGROUND
Issues with Current Inhaler Designs
Despite significant advances in new drug therapies and devices, current treatments cannot tune particle size to selectively target the route of administration. Current device design has resulted in poor patient compliance and, consequently, poor treatment outcomes.3 Existing aerosol delivery devices are based on one of the three groups:
- Pressurised metered dose inhalers (pMDIs)
- Dry powder inhalers (DPIs)
- Nebulisers.
pMDIs, or “puffers”, offer a cheap, convenient and portable solution and are widely used. They deliver aerosols in distinct, manually actuated pulses. While they appear to be easy to operate, research has shown that up to 60% of patients do not adhere to prescribed regimens and up to 90% of patients do not perform all essential steps required to use a pMDI correctly.4 For the device to be useful, patients need to be trained in, and capable of, controlling their inhalation strength and holding their breath post inhalation for 5–10 seconds. For instance, Noble (FL, US) markets “respiratory trainer” devices to teach patients how to use pMDIs effectively,5 and Adherium (Auckland, New Zealand) produces patient adherence and data management solutions, such as the Hailie platform.6 Another major challenge for patients with compromised lung functions is effectively controlling breathing patterns; there is an even more significant disease burden (as measured through disability-adjusted life years) felt by the elderly (over 65 years) and the young (below 15 years).1 These patients experience more difficulty administering medication through conventional devices due to a lack of dexterity. Incorrect inhaler techniques have not improved over the past few decades, which points to an urgent need for new approaches to delivering drugs to the lungs.7
“With PALM, drugs can be delivered in synchronised pulsed doses, uniquely combining the operational principles of pMDIs and nebulisers, and enabling a personalised aerosol delivery rate.”
A commonly identified problem among users is that they do not adhere to their treatment regimens when prescribed multiple devices, such as a preventer and reliever.8 Patients prefer the use of a single device (often a reliever) and would not use an “add-on” component (spacer) even when prescribed to do so.
DPIs are portable devices that overcome patient training and coordination issues, as they are breath activated and can deliver a higher mg/dose. However, they require a very high inspiratory pressure (approximately 4 kPa) and, consequently, are not suitable for patients with compromised lung functions.9
Unlike pMDIs and DPIs, a conventional jet nebuliser delivers a set volume of fluid at a constant rate for as long as 15 minutes without any pause. Typically, they require a source of compressed air, which makes them bulky. Ultrasonically actuated mesh nebulisers employ a vibrating perforated plate in contact with the fluid to generate a mist of droplets, which offer advantages in portability and hence have become increasingly popular alternatives. However, they have several important limitations for personalised therapies:
- The aerosol particle diameter for each drug/device pair is fixed and cannot be tuned; often specific drug formulations are associated with specific mesh designs. However, since nebulisers are manufactured independently from the formulation, patients use them with different drugs, which can lead to variations in the performance.
- Due to high fabrication costs, it is not possible to employ single-use meshes. Hence, the components need to be cleaned in between uses. Poor cleaning often hampers the performance and may result in infections.
- The existing designs involving mouthpieces avoid bulky masks but are not practical for frequent use over extended periods.
PALM – A PATENTED MICROFLUIDIC TECHNOLOGY
At the heart of PALM is a patented microfluidic aerosol generation technique. The combination of microfluidic channels, a piezoelectric driving element, associated driving electronics and various sensors are all housed within a handheld device.
The microfluidic channels are photolithographically patterned hydrophilic channels etched into a hydrophobic substrate. The piezoelectric element and driving electronics are activated by a small form factor microcontroller that triggers actuation based on measurements from an airspeed sensor. Upon actuation, a controlled volume of fluid can be drawn into the channel through a rapid capillary driven flow. The subsequent high-frequency vibration of the fluid/air interface results in a highly uniform mist of micrometre-diameter droplets, as shown in Figure 3.
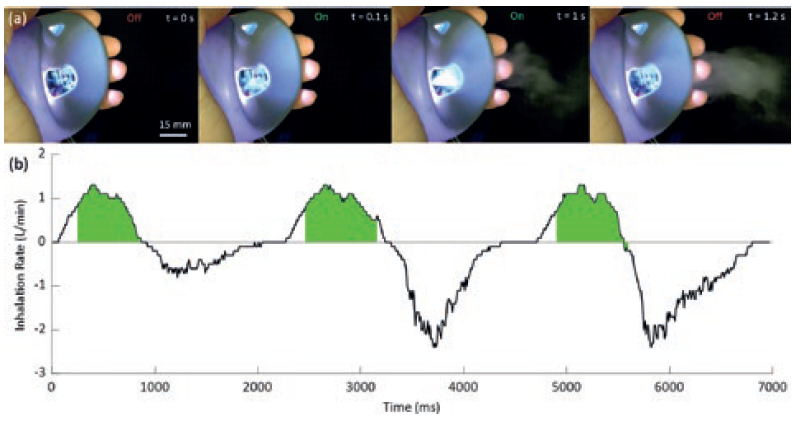
Figure 3: Example of an ejection of a mist of droplets during a 1.1 s pulse of the PALM device and a measured breathing cycle with device actuation indicated in green.
“The breathing cycles of a user are continuously monitored with airflow meters and the device is triggered when a target airflow rate is detected.”
PALM has three key advantages. Firstly, the hydrophilic channels can deliver the fluid from an arbitrary reservoir to the active region of the nebulising chip through capillary action, as shown in Figure 3. Secondly, it constrains the fluid interface to the top of the channels ensuring a very well-defined and stable interface for nebulisation. Thirdly, the new microfluidic approach can initiate and stop particle ejection in under 0.1 s. As such, with PALM, drugs can be delivered in synchronised pulsed doses, uniquely combining the operational principles of pMDIs and nebulisers, and enabling a personalised aerosol delivery rate. A comparison of the performance of PALM with a mesh nebuliser and pMDI are shown in Table 1, highlighting the high fine particle fraction (FPF) and tight size distribution.
PALM | Mesh Nebuliser | pMDI | |
Fine Particle Fraction (FPF) <5 μm |
70.86 % ± 9.11 | 64.4 % 12.2 | 52.0 % ± 2.9 |
Mass Mean Aerodynamic Diameter (MMAD) μm | 3.05 μm ± 9.11 | 3.99 μm ± 0.73 | 4.3 μm ± 0.19 |
Geometric Standard Deviation (GSD) | 1.75 ± 0.04 | 1.82 ± 0.02 | 1.75 ± 0.04 |
Table 1: Comparison of key aerosol metrics for PALM device with the commercially available Philips Innospire Go, both aerosolising salbutamol sulphate with a flow rate of 15 L/min and an Intal 1 mg pMDI containing sodium cromoglycate with a flow rate of 30 L/min.10
Synchronising Breathing Cycles with Drug Delivery
Currently, 45% of pMDI users are not able to co-ordinate their button-press and inhalation during pMDI activation correctly, and 44% do not inhale deeply or quickly enough to comply with the current correct technique.7 The PALM method of delivery is designed to remove button-press activation, instead being reactive and activated automatically by inspiration. PALM synchronises the output of medication to the inhalation of the patient, thereby acting as a “breathing device”. The breathing cycles of a user are continuously monitored with airflow meters, and the device is triggered when a target airflow rate is detected, as demonstrated in Figure 4. The device measures three breaths before calculating the target delivery window, shown here from 20% of inhalation time, until just before exhalation begins. PALM can then continue to administer medication until the total required dose is delivered.
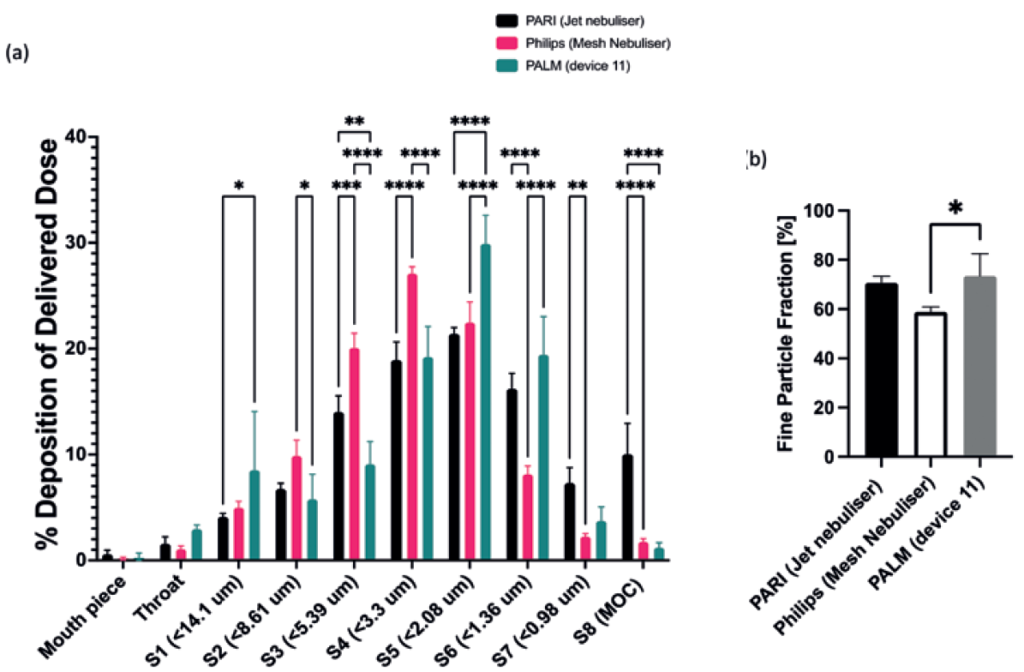
Figure 4: (A) Next-generation identification (NGI) comparison of salbutamol sulphate deposition using PALM (n=3), PARI (n=3) and Philips (n=3) nebulisers, flow rate 15 L/min. (B) Comparison of delivery device FPFs (values are means ± standard deviation, n=3). Statistical significance was determined using a two-way analysis of variance with Tukey’s multiple comparison analysis, where *p<0.033, **p<0.0021, ***p<0.0002 and ****p<0.0001.
SUMMARY
PALM combines a patented, highly responsive aerosol production approach with an airflow sensor and microcontroller to define the timing of aerosol delivery precisely. The developers have demonstrated that using the PALM device results in an improved FPF compared with typical devices currently available on the market. The developers envisage this combination of features and flexibility of dosing will improve adherence and the patient experience, while enabling the delivery of many different treatment regimes.
ABOUT THE DEVELOPMENT TEAM
Personalised Airway and Lung Management (PALM) is a world-first drug delivery technology designed by Monash University researchers. The team includes Dr Tuncay Alan and Dr Jason Brenker, microfluidic technology experts; Prof Bruce Thompson, a champion in respiratory medicine; pharmaceutical experts based at the Woolcock Institute in Sydney lead by Prof Daniela Traini and Dr Hui Xin Ong; and experts in healthcare device design lead by Prof Daphne Flynn from the Design Health Collab, also based at Monash University. The work is funded by a development grant from the National Health and Medical Research Council (NHMRC) of Australia (Grant 2000120).
REFERENCES
- “Global Burden of Disease (GBD)”. Institute for Health Metrics and Evaluation website, accessed Mar 2022.
- Usmani OS et al, “Effects of Bronchodilator Particle Size in Asthmatic Patients Using Monodisperse Aerosols”. J Appl Physiol, 2003, Vol 95(5), pp 2106–2112.
- Chapman KR et al, “Improving Patient Compliance with Asthma Therapy”. Respir Med, 2000, Vol 94(1), pp 2–9.
- Restrepo RD et al, “Medication Adherence Issues in Patients Treated for COPD”. Int J Chron Obstruct Pulmon Dis, 2008, Vol 3(3), pp 371–384.
- Reynolds J, “Best Practices and Considerations in Developing Effective Training Devices for the Pulmonary Healthcare Market “. ONdrugDelivery Magazine, Issue 96 (Apr 2019), pp 28–30.
- Jochmann A et al, “Electronic Monitoring of Adherence to Inhaled Corticosteroids: An Essential Tool in Identifying Severe Asthma in Children”. Eur Respir J, 2017, Vol 50(6), Article 1700910.
- Sanchis J, Gich I, Pedersen S, “Systematic Review of Errors in Inhaler Use: Has Patient Technique Improved Over Time?”. Chest, 2016, Vol 150(2), pp 394–406.
- Hardwell A et al, “Technique Training Does Not Improve the Ability of Most Patients to Use Pressurised Metered-Dose Inhalers (pMDIs)”. Prim Care Respir J, 2011, Vol 20(1), pp 92–96.
- Copley M, “Using Breathing Simulators to Enhance Inhaled Product Testing”. Copley Scientific white paper, Mar 2014.
- Mitchell JP et al, “Aerodynamic Particle Size Analysis of Aerosols From Pressurized Metered-Dose Inhalers: Comparison of Andersen 8-Stage Cascade Impactor, Next Generation Pharmaceutical Impactor, and Model 3321 Aerodynamic Particle Sizer Aerosol Spectrometer”. AAPS PharmSciTech, 2003, Vol 4(4), Article E54.