To Issue 139
Citation: O’Prey C, “Sustainable Design & Manufacture in Medical Devices: Where to Start”. ONdrugDelivery, Issue 139 (Oct/Nov 2022), pp 6–12.
Cormac O’Prey discusses the challenges and advantages of implementing circular-economy principles in the medical device sector, with a particular consideration of the difficulty of making remanufacturing work for high-volume, low-value devices.
INTRODUCTION
Last year, I wrote a piece in ONdrugDelivery on the drivers for sustainability in medical device design and manufacture, and an overview of some of the options available.1 Since then, in a flurry of post-pandemic activity, the medical industry has woken up to the urgent need to do something about sustainability. Some might say this is due to the sudden realisation that the UK NHS really means it when it says it will not use suppliers who do not comply with its supplier sustainability requirements. The question now being asked by drug delivery device, critical care equipment, personal protective equipment and wound care manufacturers is how to meet those requirements. Medical equipment manufacturers need to know where to start, how to measure improvements so compliance can be demonstrated, what needs to be done to the design and manufacture of devices and, most importantly, how to manage risk so that patient safety is not compromised.
“Efforts to reduce high GWP gas emissions will do little to address the issue of plastic waste from used devices, packaging and manufacturing by-products.”
In response to customer, clinician, user and regulatory demands, many medical device manufacturers are increasingly being held accountable for their plastic waste and carbon equivalent (CO2e) emissions. Many look to circular economy models for recovery and processing of used devices, but the challenge is how to make these commercially sustainable, and where to start – you start with what you can get back.
Although environmental impact has been an area of concern in business sectors, such as electronics and automotive, for many years, the medical device industry has largely been considered exempt until relatively recently. With the priority being patient safety, designers and manufacturers of medical devices have historically considered the environmental impact of their operations to be of secondary or little concern. This has changed. But how can manufacturers respond to the need to reduce the environmental impact of their products without damaging their businesses?
In a June 2022 update on a 2015 study, The Lancet found that pollution remains responsible for approximately nine million deaths per year globally.2 This makes pollution the world’s largest environmental risk factor for disease and premature death, corresponding to one in six deaths worldwide. We now know that the medical and healthcare industry is responsible for a significant part of this pollution and is directly contributing to environmental damage that results in increased human mortality rates.
If ranked alongside countries, the healthcare industry would be the fifth-largest emitter of CO2 on the planet.3 Healthcare contributes 4–5% of all global greenhouse gas emissions, with inhalers comprising a significant portion of that; in the UK, inhalers account for 3–3.5% of the NHS’s carbon footprint.4 Amanda Pritchard, Chief Executive of NHS England stated on September 30, 2021, on the NHS Public Board that “the effects of poor air quality and climate change are already being seen in our GP practices and in our hospitals, and it is absolutely right that we are part of the solution. But we can’t do this alone. It is so important that we throw down the gauntlet today to our suppliers too”. If the fundamental mission of healthcare professionals and those in the medical industry who support them is to extend life and prevent suffering, we are getting it wrong. We need to change.
WHAT IS SUSTAINABILITY – DEFINITIONS AND INDUSTRY FOCUS
In 1987 The UN World Commission on Sustainability appointed the Brundtland Commission to create what has now become widely accepted as the standard definition of sustainability – “Sustainable development seeks to meet the needs and aspirations of the present without compromising those of the future”.5 Improving sustainability and reducing the environmental impact of the medical and healthcare industry covers many approaches including, for example, improving energy efficiency and water usage. Sustainability in the context of drug delivery devices has broadly focused on two disciplines – sustainable product design and sustainable manufacture – where CO2, CO2e gas emissions and environmentally damaging material waste are minimised.
With global warming and climate change identified as the top environmental priority, the focus has been on reducing the emissions of high global warming potential (GWP) gasses. As an example, 28% of GSK’s CO2e emissions (amounting to 8.4 million tonnes) come from pressurised metered dose inhaler (pMDI) cannister propellants alone, and this is where efforts to reduce environmental impact in the inhaled medicine sector have been concentrated. However, medical device manufacturing also plays a part in reducing greenhouse gas emissions. In the US, manufacturing accounts for almost a quarter (23%) of direct carbon emissions overall, according to the US Environmental Protection Agency.6 In Europe, the situation is equally dire; the industry emits an annual total of 880 million tonnes of CO2e making it one of the largest emitters of greenhouse gases on the continent.
That said, there are options for improvement. According to the UN, an 80% reduction in CO2e emissions is achievable by adopting sustainable manufacturing.7 However, while embracing more circular manufacturing methods can help achieve reductions in GWP gasses, as well as reducing plastic waste, efforts to reduce high GWP gas emissions will do little to address the issue of plastic waste from used devices, packaging and manufacturing by-products. We need to take care of our rubbish as well.
DRIVERS BEHIND SUSTAINABILITY – WHY SHOULD WE CARE AND WHY DOES IT MATTER TO MEDICAL?
As well as the direct impact on human health, global warming and pollution from waste plastic, other factors are now driving device designers and manufacturers to take sustainability seriously, including clear messages from high-profile customers, regulatory authorities, healthcare professional groups, patients, the general public and their own staff. According to research by Oliver Healthcare Packaging, sustainability is now a strategic priority for the top 20 medical device manufacturers.
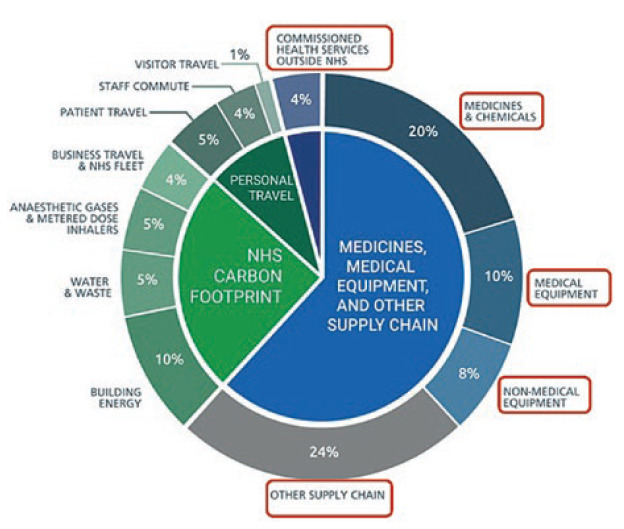
Figure 1: Breakdown of the NHS’s carbon emissions.
“Many of the techniques and strategies required to move towards
more sustainable manufacturing have already been developed in other industries or have been used in medical applications previously.”
NHS England has now identified that 62% of its carbon emissions come from the supply chain (Figure 1). As a consequence, NHS procurement practice will include net zero carbon and social value principles in all purchasing decisions. This has now been embodied in the NHS “Delivering a ‘Net Zero’ National Health Service – July 2022” report,8 which states that “all suppliers will be required to demonstrate progress in-line with the NHS’s net zero targets, through published progress reports and continued carbon emissions reporting” by 2030, as part of a structured supplier roadmap up to 2045. NHS spokespersons have further made it clear that carbon offsetting, greenwashing and commercial difficulties in meeting the requirements related to company size will not be considered valid excuses for non-compliance.
Furthermore, in the EU (and possibly in the UK), the EU Plastic Packaging Waste Directive (PPWD) will charge manufacturers of medical plastic waste at a rate of €0.8 (£0.7) per kg of nonrecycled plastic packaging. This will affect high-volume, low-value medical device manufacturers and may well require decisions about the value of used device and packaging recovery schemes to be re-evaluated.
In the US, Extended Producer Responsibility (EPR), which “places the financial or physical responsibility of packaging and products’ end-of-life on manufacturers”,9 was started in 2021 and is being rolled out across multiple states. The focus is on plastic packaging, with up to nine states expected to pass state bills in 2022 outlining EPR regulations for packaging, including Washington, California, Colorado, Minnesota, Illinois, Maryland, New York, Connecticut, Vermont and Massachusetts.10 As the legislation will affect the business case for group purchasing organisations, which collectively provide 70% of all healthcare funding in the US, and many drug delivery devices are identified as “secondary packaging”, it remains to be seen how this legislation will be applied in the global medical device industry.
In conclusion, the need for medical device manufacturers – including drug delivery device and single-use device (SUD) manufacturers – to engage with sustainability in reducing their plastic waste, as well as their CO2 emissions, is compelling and urgent. The question is – what to do about it?
SUSTAINABLE DESIGN AND MANUFACTURE IN MEDICAL – WHAT TO DO AND WHERE TO START
The good news for medical device manufacturers, as indicated earlier, is that many of the techniques and strategies required to move towards more sustainable manufacturing have already been developed in other industries or have been used in medical applications previously. Indeed, many procedures for reusing surgical instruments were made standard practice decades ago, and some medical device manufacturers have been using circular manufacturing practices for economic reasons for many years.
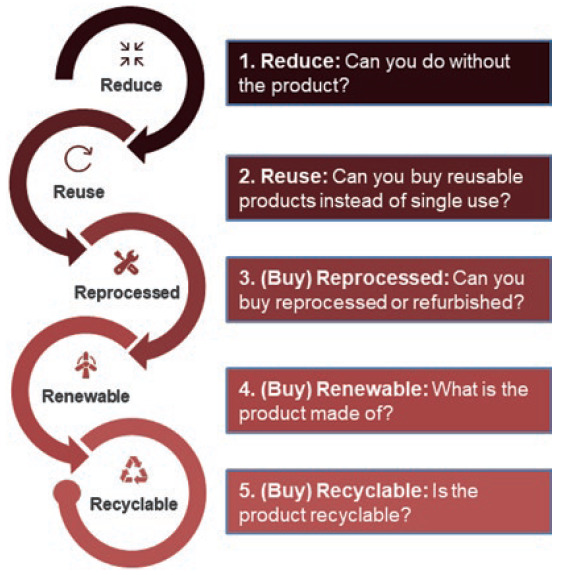
Figure 2: The 5Rs approach for reducing carbon emissions.
The medical device industry is, by nature and for good reason, intrinsically risk averse. Adopting and adapting proven models from other sectors and from old medical device management procedures therefore offers advantages in reduced risk, lower costs and shorter adoption timeframes. The NHS has released “How-to Guides” for trusts to help identify improvement strategies, including the “5Rs” (Figure 2).
Sustainable manufacturing models such as the “Circular Economy” model promoted by the Ellen Macarthur Foundation and the “Design for Manufacture, Assembly, Disassembly and End of Life Processing (MADE)” model described in BS 8887-1:2006, provide a range of options for reducing the environmental impact of manufacturing operations.11,12 The BS 8887 TPR1/7/5 subcommittee created in 2021 now specifically looks at standards and best practice for medical devices, supported by medical device manufacturers, designers, researchers and, crucially, regulatory authorities, as well as customer organisations.
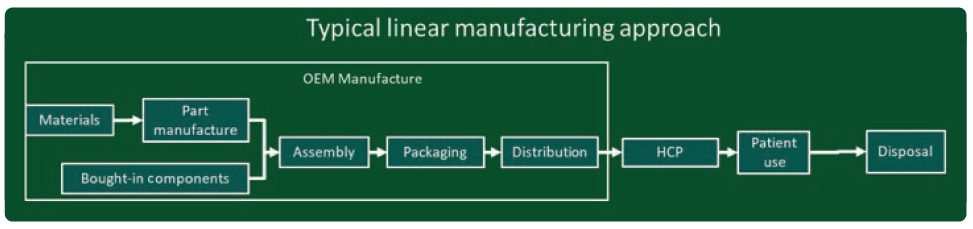
Figure 3: Diagram of a linear manufacturing model.
In both these models, businesses are encouraged to move from traditional “linear” manufacturing (Figure 3) to a more “circular” model (Figure 4). In traditional linear manufacturing, products are manufactured, shipped, distributed to users (patients) and disposed of. No used products are recovered and what happens to the used products and packaging is not considered to be the concern of the manufacturer.
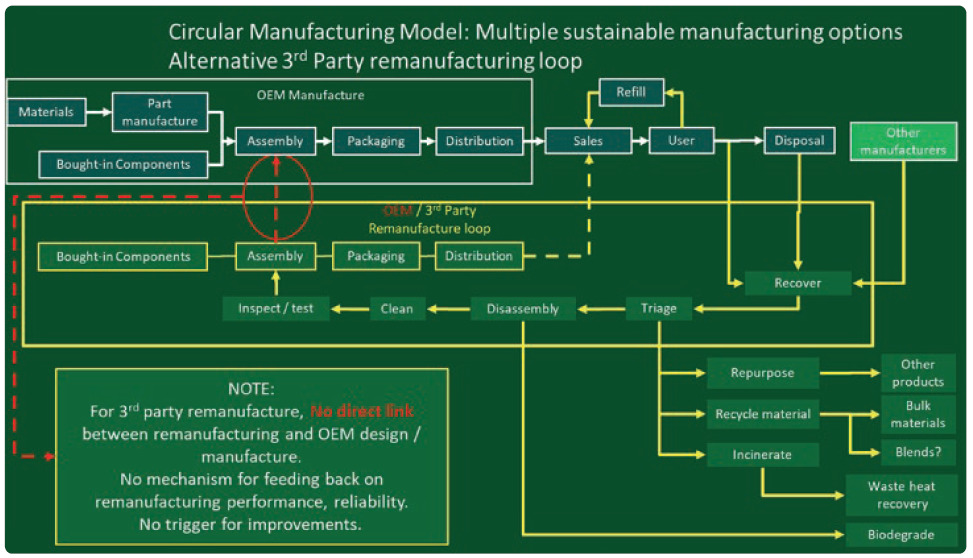
Figure 4: Diagram of a circular manufacturing model.
In a circular model, used devices are recovered and processed in various ways that reduce their environmental impact. So far, these have tended to be material recycling schemes to keep devices out of landfill, such as the GSK and Novo Nordisk recycling schemes,13 but can also include secondary use in other applications, refurbishment and resale, life extension and even composting components made from biopolymers. It is a more complex model with multiple options and routes to consider, which can be off-putting for some manufacturers.
The preferred route, however, is remanufacturing. Indeed, the NHS’s “Device Remanufacture ‘How To’ Guide: Medical Devices” focuses on remanufacturing as one implementation approach of the circular model, and the Ellen Macarthur Foundation recommends remanufacturing as the least environmentally impactful, but most commercially viable, route for used devices.11 Remanufacturing returns used or partially used devices to the market in “at least as good as new” condition with accompanying warranties and at the equivalent price of a new device. The classic circular economy model describes used devices returned to an “as-new” condition. However, an alternative “spiral” implementation of the circular model recognises the need for manufacturers to keep their recovered products current (Figure 5).
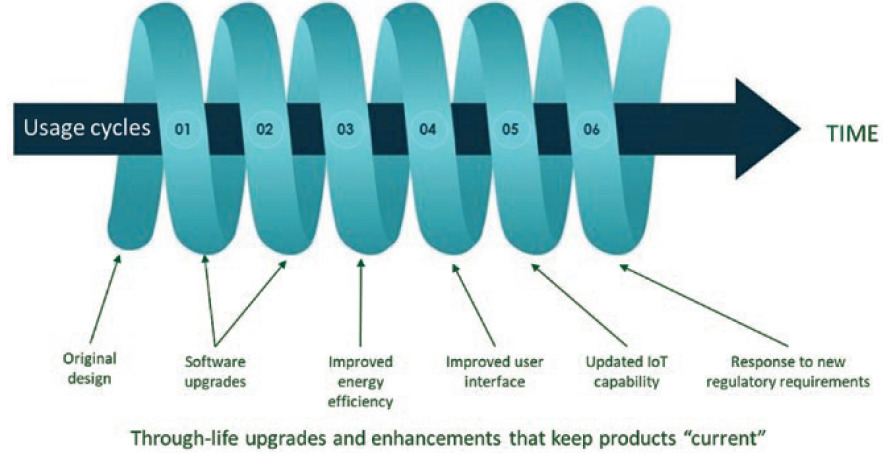
Figure 5: Some medical device developers may prefer a spiral economy approach to a circular one.
During remanufacturing, devices must be cleaned and repaired to an as-new condition, but as-new may not be what the market needs if sufficient time has passed to render the product old-fashioned or obsolete. Designers must leave room for new technology or changes in aesthetics to be accommodated in future cycles. Nokia has successfully followed this approach in the telecommunications industry for many years.14
“Remanufacturing can look attractive for manufacturers in principle because, unlike recycling schemes where any value embedded in used devices is largely written off, significant “residual value” can be recovered from some used devices.”
Remanufacturing can look attractive for manufacturers in principle because, unlike recycling schemes where any value embedded in used devices is largely written off, significant “residual value” can be recovered from some used devices. This can offset new product manufacture costs by reducing the number of new components that must be supplied and assembled. Reductions in transportation and disposal costs, as well as the aforementioned 80% reduction in CO2e emissions, can be achieved, removing a sales barrier to some customers. The key to the commercial viability of this process, however, is for the residual value recovered to be worth more than the cost to recover it. This is where many manufacturers of low-cost or low-residual value devices are currently stuck.
Lifecycle analyses and sales incentivisation programmes based on existing linear economy models typically only look at provision of new products to the market. Circular/spiral models require a more complex understanding, often going back further to include assessment of the environmental impact of extraction and material refining operations, as well as looking ahead at post-use impact. Furthermore, the implementation and integration of complex and unknown recovery, processing and re-introduction schemes to allow recovered components to be used in remanufactured products would affect businesses, suppliers and customers, as well as represent a significant risk of disruption (Figure 6).
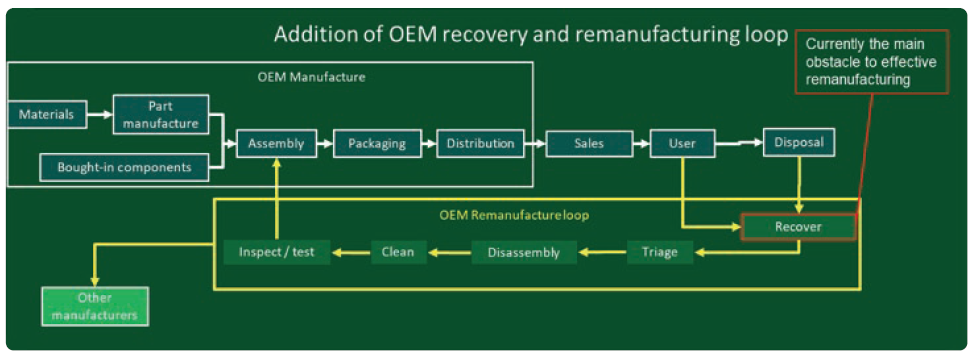
Figure 6: Diagram of a circular manufacturing model based on remanufacture by the OEM.
Established remanufacturing schemes in the medical sector have so far been largely limited to low-volume, high-value equipment, such as General Electric’s medical imagers.15 The labour-intensive processes required can be expensive, slow and non scalable, which may not be a serious issue for small quantities of manually assembled multimillion-dollar imaging equipment, but is not viable for high-volume and relatively low-value products, such as disposable SUDs. For remanufacture to be considered a serious proposition in these areas, an approach more like design for manufacture and assembly (DFMA) is required.
Research into remanufacturing automation is being carried out by the University of Birmingham and Wuhan University, supported by Chinese national standards bodies in collaboration with BSI, and is reported on annually at the International Workshop on Autonomous Remanufacturing (IWAR). Drug delivery device remanufacture needs to operate at volume, speed, reliability and, crucially, low processing costs to pay for itself.
“Successfully integrated product designs and manufacturing solutions have been established through the familiar DFMA approach, but now design for remanufacture must also be considered.”
RECOVERY – THE ELEPHANT IN THE ROOM
A significant challenge for drug delivery device remanufacturing is how to achieve useful device recovery rates (how many you can get back) and recovered device yield rates (how many of those are of any use). Recovery of hospital-based devices, such as surgical equipment, has seen success due to its closed-loop nature, but devices released to the public outside of clinical environments are particularly problematic. Novo Nordisk has admitted problems to date13 and, in September 2021, GSK reported that, after 10 years in operation, its pMDI recycling scheme only had a 0.4% recovery rate. The most sophisticated remanufacturing operations will fail if they have no used products to remanufacture, which has been an intractable issue for most organisations attempting this approach with medical devices.
Incentivising patients and users to return used devices is difficult. With low residual- value devices, deposit return schemes (DRSs), where users are offered cash rewards for returning devices, need to offer a sufficient amount for users to bother. If this is more than the residual value of the device, the scheme will lose money – assuming the device is actually usable at all. If patients are asked for an additional deposit at the dispensing point that will be returned when a used device is returned, treatment is no longer free at the point of use, with all the political problems that implies. Insisting that patients return used devices in exchange for new ones raises the possibility of doctors refusing life-critical treatment to patients who lose or forget their old devices. The consequences of that are as obvious as they are unacceptable. True change requires a change in patient culture to drive an attitude that throwing your used device away is no longer acceptable. While history shows cultural change can be both difficult and slow, it could perhaps be achieved by helping patients understand the environmental and financial impacts of not complying with sustainable practices. This could be combined with schemes to make return as convenient as possible and by providing benefits to patients, such as helping to “de-clutter”. Pilot schemes in the NHS to encourage the return of walking aids may offer some useful learning points.
Successful recovery schemes for high-volume, low-value products, such as plastic water bottles, coffee pods and aluminium drinks cans, have been developed in other industries, although their success rates are still in question. Approaches such as reverse vending machines,16 DRSs and loyalty card points schemes are established in the food packaging industry. These have the potential to be adapted for use in medical devices, and new technologies, including unique low-cost part-marking solutions from Polytag, offer potential solutions for the tricky EU Medical Device Regulations (MDR) requirement to ensure the number of reuse cycles for each component are tracked and limited. Automated used-product picking systems can identify and pick devices from refuse streams in real time and can carry out superficial inspection and sorting activities. As with remanufacturing operations, used device recovery must be automated, scalable, reliable and cost effective.
IMPLEMENTING REMANUFACTURING
For organisations looking to implement a circular or spiral economy model, assuming that useful quantities of usable used devices can be recovered, the question remains – where to start? It is a complex and rapidly-evolving landscape. Successfully integrated product designs and manufacturing solutions have been established through the familiar DFMA approach, but now design for remanufacture must also be considered. Designers do not yet know what this process looks like, in no small part because it is still evolving. Designers also need to understand what the remanufacturing process needs from their designs, but this process does not yet exist. Ideally, designers need to understand what the different routes for each recovered component are likely to be so that they can optimise the design accordingly.
Experience from other industries has shown that 100% component reuse is unrealistic, so designers need to differentiate and plan to separate the remanufacturable “core” from components that can be discarded. This will require a change in requirements specifications and design verification testing procedures for multiple-lifetime parts, which will be different from the specifications for disposable items. Counterintuitively, the part count may need to go up to facilitate the separation of worn and contaminated features from reusable ones that were previously considered the same part.
The amount of plastic used in some parts may also need to increase to improve long-term robustness, and different materials may need to be considered. Increasing the amount of plastic, however, only becomes an issue when devices are discarded rather than reused, and a primary aim of remanufacturing is to reduce the number of discarded devices. Disassembly will also need to be considered, possibly using automation, but in such a way that patient safety is not compromised. Designs must also mitigate against the risk of users accidently taking devices apart.
So where do designers start? They start by looking at existing used devices. As stated earlier, without an effective device recovery scheme, the entire remanufacturing approach is pointless, so establishing an effective used device recovery scheme – at least as a pilot or feasibility study – must come first. This provides designers with evidence of real-life degradation in existing mechanisms that can be used as a basis for more robust remanufactured product designs. Recovered devices reveal how they have been used, abused, damaged, worn, contaminated and broken.
This, in turn, informs the design process as to which elements in the design need to be replaced and which need to be reinforced to provide reliable long-term use as part of the remanufactured core (Figure 7). This process can then inform the critical risk analysis required by the regulatory authorities, backed by physical evidence, and has the additional benefit of providing increased levels of post-market surveillance data, assuming this data makes its way back to the original equipment manufacturer (OEM). Indeed, this is something called for in recent changes to the EU MDR by the EU Medical and Healthcare Regulatory Authority.

Figure 7: The route to establishing a remanufacture-based paradigm starts with recovery of existing devices for analysis to inform future designs.
Improvements in device reliability can reasonably be expected to result from these insights. If “smart” devices are recovered, there is the further possibility of adding self-diagnostic functionality whereby the device can directly communicate with the remanufacturing facility to tell remanufacturers what has happened to it, how much it has been used, if it has suffered any serious abuse and therefore whether or not it is worth reprocessing.
Once all these factors have been assessed, the design can feed into strategies for:
- Reprocessing and remanufacturing, including what components need to be disassembled
- Disposal, remanufacture, repair and upgrades
- Decontamination and sterilisation processes
- Inspection and test requirements
- Considering variabilities
- Process automation.
The objective will be a design that is optimised for multiple usage cycles, with an evidence-based risk management strategy, and a manufacturing and remanufacturing solution able to deliver at maximum yield and minimum cost.
FUTURE DEVELOPMENTS
There is more detail to consider – including how to manage legacy components, design verification testing and accelerated life testing to cover extended product lifetimes – but there are also hidden benefits. Recovery of used smart devices can give access to device performance and anonymised patient usage data, improving their value as diagnostic tools and providing valuable clinical insights into patient population behaviour. Remanufacture of smart drug delivery devices can improve affordability without sacrificing profitability by amortising high manufacture and component costs over multiple usage cycles. Additionally, as stated earlier, incorporating a self-diagnostic capability in smart devices could improve yield and quality in remanufacture by enabling devices to communicate directly with automated remanufacturing facilities.
Longer term, redefining the relationship between manufacturer and patient to revolve around regular return, remanufacture and upgrade of familiar devices could improve patient confidence and establish a “lock-in”, where patients are unlikely to switch to a different supplier. Some manufacturers may even consider transitioning to a “product-as-a-service” model, where ownership of the device remains with the manufacturer and the user pays for the use of the device as a service – suppliers maximise the value of their investment by keeping items in circulation while continuing to collect revenue for their use. Examples from other industries include the automotive sector, where 82% of new cars are leased in the UK using a similar business model.17
The refurbished medical equipment market was valued at US$9.82 billion (£8.86 billion) in 2019 and is expected to reach $23.91 billion by 2026 with a compound annual growth rate of 11.80% over the forecast period.18 Sustainable manufacturing in the medical sector is a serious business – the medical device business should take it seriously.
REFERENCES
- O’Prey C, “Sustainability, Waste & Remanufacturing in the Medical Sector”. ONdrugDelivery, Issue 126 (Oct/Nov 2021), pp 12–16.
- Fuller R et al, “Pollution and Health: A Progress Update”. Lancet Planet Health, 2022, Vol 6(6), pp E535–E547.
- “Greener NHS Campaign to Tackle Climate ‘Health Emergency’”. UK NHS press release, Jan 2020.
- Tennison I et al, “Health Care’s Response to Climate Change: A Carbon Footprint Assessment of the NHS in England”. Lancet Planet Health, 2021, Vol 5(2), pp E84–E92.
- “Sustainability”. UN web page, Accessed Sep 2022.
- Lundstedt M, “From Remanufacturing to Recycling: How Manufacturing Can Raise the Bar on Global Climate Goals”. World Economic Forum, Jun 2021.
- Nasr N et al, “Re-defining Value – The Manufacturing Revolution. Remanufacturing, Refurbishment, Repair and Direct Reuse in the Circular Economy.” UN Environment Programme report, 2018.
- “Delivering a ‘Net Zero’ National Health Service”. UK NHS report, Jul 2022.
- Fording A, “Extended Producer Responsibility Legislation: 2021 State Trends”. FiscalNote, Oct 2021.
- “Extended Producer Responsibility for Packaging in the United States”. Valpak, May 2022.
- “What is a Circular Economy?”. Ellen MacArthur Foundation webpage, Accessed Sep 2022.
- “BS 8887-1:2006 – Design for Manufacture, Assembly, Disassembly and End-Of-Life Processing (MADE) General Concepts, Process and Requirements”. BSI, Oct 2006.
- “Can You Recycle an Insulin Pen?”. Novo Nordisk, Jan 2022.
- Tanskanen P, “Make Do and Mend – Circularity Comes Full Circle”. Nokia, Jan 2020.
- “Refurbish, Reuse and Refresh: 3 Ways to Extend the Life of Devices Used to Extend Patient Lives”. General Electric, Apr 2022.
- “Reverse Vending Machines for Bottle and Can Recycling”. Tomra webpage, Accessed Sep 2022.
- Edwards J, “The UK Car Business Has ‘Exactly the Same Problems’ As the Mortgage Market 10 Years Ago, According to Morgan Stanley”. Insider, Aug 2017.
- “Refurbished Medical Equipment Market – Global Industry Analysis, Size, Growth, Share, Trends, Segmentation, Opportunity and Forecast 2020 to 2025”. Medgadget, Jul 2020.