To Issue 164
Citation: Allmendinger A, Adler M, Delauney C, Mahler H-C, “Technical Challenges During the Development of On-Body Injectors”. ONdrugDelivery, Issue 164 (Sep 2024), pp 14–20.
Andrea Allmendinger, Michael Adler, Carole Delauney and Hanns-Christian Mahler of ten23 health provide an overview of large-volume wearable injection devices for the subcutaneous delivery of biologics and discuss the technical challenges associated with the development and manufacturing of these drug/device combination products.
Today, biologics are increasingly being administered via subcutaneous (SC) injections. As such, demand has grown for high-volume delivery systems capable of injecting volumes larger than 1 mL and 2.25 mL, which are the typical container formats used in autoinjectors. Several factors are contributing to this trend, including therapeutic advantages, such as self-administration or caregiver assisted administration, that offer increased convenience in home or office settings and reducing hospital visits. This also reduces overall healthcare costs and offers scheduling flexibility – minimising capacity constraints.
Unlike intravenous (IV) injections (with 100% bioavailability), SC administration typically results in lower bioavailability – around 60–80% for monoclonal antibodies (mAbs).1 Consequently, SC delivery often requires slightly higher doses than IV delivery and “fixed doses” are typically preferred. Traditionally, SC injections were believed to be limited to volumes up to 1 mL. However, the trend towards SC injections for “high-dose” products such as antibodies (in the range of up to 1,000 mg per dose per patient) has led to the development of highly concentrated formulations (>180 mg/mL), which has fuelled discussions about the maximum injection volume for SC injections to achieve target doses.
Temporary modifications in the SC tissue by recombinant human hyaluronidase helped with the injectability of volumes greater than 10 mL. However, increasingly, there have also been explorations of SC injection volumes beyond 10 mL (up to 25 mL), without the use of enzymes or other permeation enhancers.2
In addition, a paradigm shift is ongoing, exploring the adaptability of low-to-moderate-concentration drugs in high-volume formulations. This offers an alternative to high-concentration, low-volume SC delivery, avoiding high-concentration formulation challenges related to stability, manufacturability and administration (e.g. due to viscosity) and reducing the development risks associated with high-concentration formulations.
The development of these low-to-moderate-concentration high-volume SC injection products is, of course, further enabled by the availability of platform-based, typically ready-to-use (RTU) –i.e. pre-assembled, prefilled and preloaded – large-volume SC injection devices, such as on-body injectors (OBIs). These devices are typically attached to the body prior to injection and are also referred to as patch injectors or wearable injectors.
MARKET OVERVIEW
The market in this space is still relatively new, with most technologies still in development and only a few commercial drug products using OBIs having been approved by health authorities. Between 2015 and 2023, there have been six wearable drug delivery products that have achieved market approval using devices from Insulet (Acton, MA, US), West Pharmaceutical Services, Enable Injections and LTS Lohmann, with the rate of releases accelerating in the last three years:
- 2015 – Omnipod, Insulet: Neulasta® (pegfilgastrim in 0.6 mL prefilled syringe) – Amgen
- 2016 – SmartDose, West: Repatha® (evolucumab in 3.5 mL cartridge) – Amgen
- 2022 – SmartDose, West: Skyrizi® (risankizumab in 3.5 mL cartridge) – AbbVie
- 2022 – SmartDose, West: Furoscix® (furosemide in 10 mL cartridge) – SC Pharmaceuticals
- 2023 – enFuse, Enable: Empaveli® (pegcetacoplan in 20 mL vial) – Apellis
- 2023 – Sorrel, LTS Lohmann: Udencya® (pegfilgrastim-cbqv loaded by transfer syringe) – Coherus.
West’s SmartDose is user-loaded with a prefilled 3.5 or 10 mL polymer cartridge, LTS Lohmann’s Sorrel is user-loaded using a transfer syringe (in the commercially approved configuration) and Enable’s enFuse is user-loaded with either a transfer syringe or a prefilled standard glass vial.
Device selection depends on a variety of interdependent factors, including the target product profile (TPP) in relation to the user population (e.g. injection volume, injection time, patient capabilities); product properties, such as viscosity, related to device performance, functionality and compatibility with the primary packaging container (e.g. stability); quality and regulatory aspects related to device certification, cost of goods, technology maturity and supply chain security (e.g. device and primary packaging suppliers); and the availability and capabilities of manufacturing partners (e.g. drug product fill-finish manufacturing processes and facilities). Additionally, in the light of targeted reductions in Scope 3 greenhouse gas emissions for sustainable product design, there is increasing interest in recyclable and reusable solutions.
Especially for new devices or primary packaging containers, a close working relationship between the involved parties is a must to streamline timelines and potential troubleshooting activities. Establishment of a proactive partnership between device providers and CDMOs to provide final product development and manufacturing (fill-finish) will be essential to optimise GMP manufacturing timelines and secure fast-to-market access.
To give an overview of current technologies, Table 1 provides a non-exhaustive list of currently available wearable SC injection devices, including their volume delivery range, mechanism of delivery, primary packaging material and other features (e.g. smart connection, reusable options).
Company | Product | Target Delivery Volume |
Delivery Mechanism | Primary Packaging Formats |
Loading | Additional Features |
BD | Libertas™ | 2–5 mL and 5–10 mL |
Mechanical spring-based |
Glass container | Prefilled container, preloaded |
– |
BD | Evolve™ | Up to 3 mL | Electromechanical | Glass container | User-loaded | Catheter-mediated injection up to three days: Connected device; Programmable electronics |
CCBio | Felice Dose | 20 mL | Electromechanical | Polymer mini-bag | Prefilled container, preloaded |
– |
Enable Injections | enFuse®* | 10–50 mL | Pressure-driven | Vial, transfer syringe, dual vials (lyo/diluent with automated reconstitution) |
User-loaded (with prefilled container or transfer syringe) |
Connected device |
Gerresheimer | SensAir® On-Body Delivery Device |
10–20 mL | Pressure-driven | Glass cartridge | User-loaded (with prefilled container) |
Connected device |
LTS Lohmann | Sorrel™ platform* | 1–50+ mL | Electromechanical | Glass vial or cartridge |
Prefilled container, preloaded |
Software-controlled injection; Connected device |
Nemera | Symbioze® | Up to 20 mL | Electromechanical | Glass cartridge | Prefilled container, preloaded |
Sterile fluid path system; Connected device; Re-usable and rechargeable electromechanical part |
Sonceboz | LVI-V & LVI-P™ | 1–20mL | Electromechanical (GentleTouch™ piston pump) | Vials (V); Glass cartridge (P) |
Prefilled container, preloaded (LVI-P) or user-loaded (LVI-V) |
Multi-Day, dual-cartridge or automatic reconstitution options; Bridging from V to P formats, facilitating clinical bridging and lifecycle management; Applys sustainable 4R model (Reduce, re-use, replace, recycle) |
Stevanato | Vertiva® | 3+ mL | Magnetically coupled drive mechanism | Glass cartridge | Prefilled container, preloaded |
Connected device; Programmable electronics; Re-usable parts |
West Pharmaceutical Services | SmartDose®* | Up to 3.5 L or 10 mL |
Electromechanical | Polymer cartridge | User-loaded (with pre-filled container) |
– |
Ypsomed | YpsoDose | 2–10 mL | Electromechanical | 10 mL cartriQ® (SCHOTT) glass cartridge |
Prefilled container, preloaded |
Sterile fluid path system; Programmable electronics |
Table 1: Overview of OBIs based on publicly available information. (*commercially launched version)
The development of OBIs presents a variety of technical challenges. The challenges related to the design and engineering of the device or the adhesive patch are not discussed in this article, instead, it aims to review quality and safety-related aspects, such as compatibility and performance related to the interplay of the product, primary packaging container and device. This includes manufacturing challenges from a drug product development perspective, highlighting the necessity of integrated drug product development.
“Ensuring optimal functionality and performance in drug delivery systems requires careful consideration of device design and primary packaging selection, dependent on the viscosity of the drug product.”
FUNCTIONALITY AND INJECTION TIME
Ensuring optimal functionality and performance in drug delivery systems requires careful consideration of device design and primary packaging selection, dependent on the viscosity of the drug product. The viscosity of the injected solution, especially at high protein concentrations, presents significant challenges related to injection time, given the typical usage of 27G and maximum 25G needles for SC injections.
According to the Hagen-Poiseuille equation, the injection time is proportional to the viscosity, which exponentially increases for highly concentrated protein formulations, dependent on the formulation’s composition.3 The inner diameter of the needle plays a role in the injection force to the power of four. Note that the inner diameter of injection needles of the same nominal G values may differ for different providers. Another consideration is that larger administration volumes require larger primary packaging container diameters, with the injection force proportional to the radius squared.
Different mechanisms are employed to address these challenges, aiming to balance injection time and patient convenience, comprising mechanical and electromechanical systems (cartridges) and pressure/collapsible container-based systems (vials and cartridges). The advantage of using vials is that there is no requirement to change the primary packaging container type after early clinical Phase I/II studies – only adjustments of container size and fill volumes – incurring fewer technical challenges compared with any change in primary packaging container formats. Pressure-driven systems must be capable of consistently delivering the intended dose volume for a given viscosity in a given injection time, ideally with minimum product loss.
For containers such as cartridges, functionality is typically characterised by testing the break-loose and glide forces, in addition to injection time. Functionality depends on the selection of the primary packaging components, including the container body (glass/polymer), inner surface (e.g. type, quality and quantity of lubrication) and stoppers (including their potential lubrication). Depending on the manufacturing process of the supplier, lot-to-lot variability of lubrication of the containers and/or stoppers, lubrication distribution during ageing and compatibility of lubrication with the drug product during storage and transport must be considered. Lubrication and fit of container closure system components may also determine the product’s manufacturability (fill-finish), such as for stopper setting.
“The compatibility and stability of a product formulation with the primary container closure system during storage, as well as in-use stability during delivery, is essential.”
PRODUCT COMPATIBILITY AND PARTICULATE MATTER
The compatibility and stability of a product formulation with the primary container closure system during storage, as well as in-use stability during delivery, is essential. The extractables and leachables profile must be assessed and the container closure integrity at the intended storage conditions must be adequately characterised and ensured. The inherent properties of the containers, such as the oxygen permeability of polymer containers, must be studied during drug product development for oxidation-sensitive molecules. In the case of coated containers, the homogeneity and integrity of the coating must be assessed and ensured over the product’s shelf life.
Additionally, the impact of lubricants, such as silicone oil, has to be considered. Silicone oil (or other lubricants) might migrate into the drug product over storage time and also shed from the cartridge during administration – entering the drug product detected as sub-visible particles during in-use testing. Testing for visible and sub-visible particles is a compendial requirement for parenteral products, with defined limits that are set out in the following pharmacopeial chapters:
- Sub-visible particles:
– USP <787> (low-volume method for biologics)
– USP <788>
– Ph.Eur. 2.9.19
– JP 6.07 - Visible particles:
– USP <790>
– USP <1790>
– Ph.Eur. 2.9.20
– Ph.Eur. 5.17.2.
Generally, these monographs define the requirements for particles, which can originate from different sources, and methods for particle testing and characterisation. The filled drug product must meet the compendial requirements for both sub visible and visible particulates. To note, the particulates eventually found in the final drug product is the sum of all particles originating from the drug product formulation (and any stability-dependent increase in particulates during storage, transport or administration), primary packaging (e.g. contamination of RTU primary packaging) and the manufacturing process, equipment and extrinsic sources.
RTU Primary Packaging Components
Primary packaging components for OBIs, such as cartridges and elastomeric closures, are mostly supplied as RTU products – washed, sterilised, pre-lubricated (e.g. siliconised) and depyrogenated by the supplier. Every particle attached to an RTU primary packaging component will end up in the drug product as there is no particle removal step during the drug product manufacturing process when using RTU components. Hence, any contaminants in RTU primary packaging can – and will – impact the yield of the filled drug product after 100% visual inspection.
As a result, adequate control of particle contamination of primary packaging components relies on the process capability to remove particulates during the manufacturing process and the control strategy by the respective supplier of primary packaging components. Material properties may impact the ability to minimise and control particulate contamination in RTU primary packaging.
Control Strategy for Primary Packaging Components
The current pharmacopeial methods and limits for visible and sub-visible particulates referenced above apply to drug products, however they do not apply to primary packaging components.
Cartridges and Vials
Visible particulates in empty containers such as cartridges must be minimised, or avoided entirely, as they can lead to contamination of the final filled drug product. However, they are often more difficult to detect in filled containers, where visible particulates are typically mobile. Furthermore, the methods used by suppliers for visual particle inspection of RTU containers are not standardised and their sensitivity may be inferior compared with the pharmacopeial methods used for drug products. In addition, there are no standardised acceptance criteria or limits for visible particulates in RTU cartridges (Table 2).
Given the probability of detection of visible particles using the pharmacopeial method for visual inspection, dependingon the type of container and the capability of the operator (Figure 1), visible particles as small as 50 μm may be detectable in final drug products.4 As a result, in a worst-case scenario, single visible particles larger than 50 μm coming from RTU containers could lead to a 100% rejection rate for the entire batch of drug product.
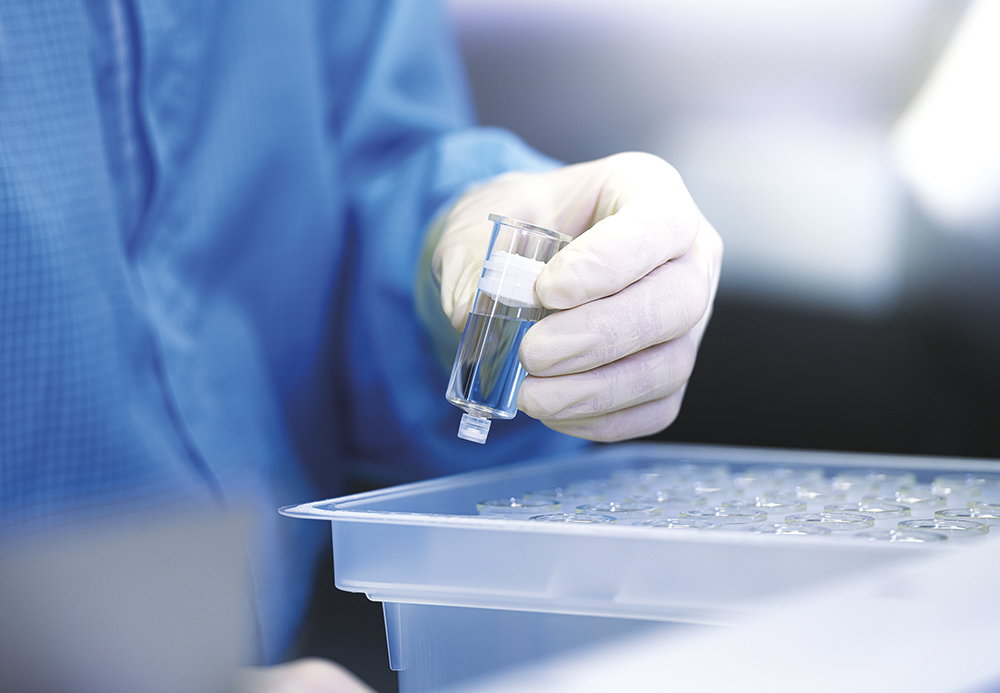
Figure 1: Inspection of drug products for visible particles.
Limits for sub-visible particulates in empty containers have been arbitrarily set to ≤600 particles per container for particles ≥10 μm, and ≤60 for particles ≥25 μm according to ISO 21881:2019 (Table 2), which is 10% of the pharmacopeial limits for filled, sterile, small-volume parenteral drug products.
ISO standard | Title | Test for visible particulates | Test for sub-visible particulates |
ISO 8871-3:2003 | Elastomeric parts for parenterals and for devices for pharmaceutical use – Part 3: Determination of released-particle count | Enumerate >25 μm; no acceptance criteria or limits |
Count ≥2 μm; no acceptance criteria or limits |
ISO 21881:2019 | Sterile packaged ready-for-filling glass cartridges |
No test | ≤600 particles/container for ≥ 10 μm; ≤60 particles/container for ≥ 25 μm |
ISO 11608-03:2022 | Needle-based injection systems for medical use – Requirements and test methods – Part 3: Containers and integrated fluid paths |
Visible particulates >150 μm; no acceptance criteria or limits |
Enumerate sub-visible particulate matter content; no acceptance criteria or limits |
Table 2: Overview of ISO standards related to the testing for visible and sub-visible particulates in combination product components.
Elastomeric Closures
A standardised ISO test method for elastomeric closures is available that includes testing for visible and sub-visible particulates (Table 2). However, the sensitivity of the test method can be questioned, due to the number of stoppers sampled being defined by the surface area in the method. Furthermore, no limits have been defined or suggested for visible and sub-visible particulates.
In summary, the industry lacks standardised test methods and acceptance criteria for testing and controlling sub-visible and visible particulates in RTU primary packaging components such as cartridges, syringes and elastomeric closures. It is therefore important to understand the process capabilities and control strategies of suppliers, as well as the lot-to-lot consistency of containers and elastomeric closures provided by suppliers, and tightly link it to the final drug product requirements, as per quality-by-design considerations.
MANUFACTURING
Drug Product Filling
The selection of an OBI and its primary packaging container involves several critical considerations besides product-related decisions. Attention must be paid to whether existing manufacturing facilities and lines are capable of filling and stoppering the container, or if a new facility or the selection of a dedicated CDMO for drug product fill-finish operations is necessary (Figure 2).
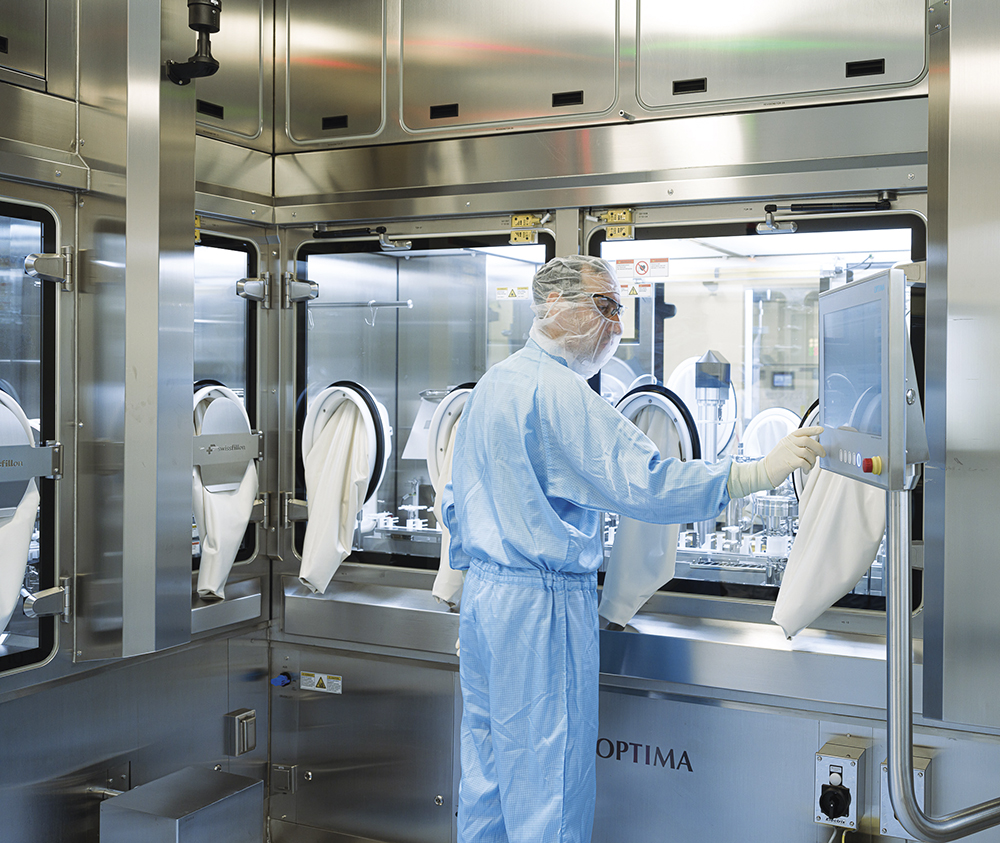
Figure 2: Operator at ten23’s filling line in Visp, Switzerland.
Several factors must be taken into account, including the availability of format parts, the ability to fill a certain viscosity range, the process knowledge and expertise of suitable stopper setting processes and the filling pump technology. Manufacturing process unit operations can lead to adverse product quality impacts and the filling accuracy and presence of headspace may impact the dosing accuracy of the device.
The stoppers used in OBIs can sometimes be custom-designed to meet specific requirements. During the stopper setting process, several issues can arise that may affect container closure integrity (CCI). One common problem is “tilting”, where the stopper does not move or sit properly during setting. Additionally, depending on the type of stopper and the manufacturer’s expertise, wrinkles in the stopper coating can occur.
Another essential aspect when filling the primary packaging container for OBIs is the presence of an air bubble, or “headspace”, in the OBI container – it is crucial to select a partner capable of filling without air bubbles. It is crucial to determine whether the stopper will sit directly on the solution, thereby avoiding headspace, or if headspace will be present. In the presence of headspace, any movement of the stopper, such as during assembly or air transport, must be carefully evaluated.
To summarise, selecting an OBI and deciding on its filling and assembly location requires detailed planning to ensure successful implementation.
Sterile Fluid Path
It is important to consider how the fluid path – from the container to drug product withdrawal to administration – is designed to ensure protection from potential environmental microbiological contamination. Some devices use specific technologies to ensure that sterility of the fluid path is maintained. Of course, the actual risk of not having a fully protected sterile fluid path has to be evaluated in detail as, at the point of drug product withdrawal, the outside of the stopper will not be sterile, only disinfected (e.g. with an isopropanol wipe). It may be theoretically possible to develop and commercialise devices without full sterile fluid path protection, based on adequate risk assessments and risk management, and in close alignment with health authorities. In such cases, device assembly may have to be performed under clean room conditions – in Class D or Class C, or even Class A under laminar flow – to minimise potential contamination of the outside product packaging during assembly. This assumes that the device and secondary packaging ensure protection against subsequent environmental contaminations.
CONCLUSION
Successful development of large-volume SC injection devices relies on making wise choices regarding the device technology and development and manufacturing partners. Optimal partners should have capabilities for integrated development, fostering collaboration between product development and manufacturing teams. Early involvement and joint discussions with primary packaging suppliers, device manufacturers, fill-finish facilities and drug product developers may enable synchronised strategies, aligned specifications and holistic troubleshooting, which can save time, costs and resources while preventing errors. Continuous communication is imperative for project success.
A CDMO such as ten23 health is appropriately positioned to overcome the challenges of large-volume injection devices. The company offers integrated development of formulation services, analytical development and product characterisation, as well as support for device selection and testing, drug-device integration and drug product filling process design and characterisation. It also provides drug product manufacturing of complex and high-precision containers at its GMP fill-finish facility and quality control release and stability testing services.
REFERENCES
- Datta-Mannan A et al, “Influence of physiochemical properties on the subcutaneous absorption and bioavailability of monoclonalantibodies”. mAbs, 2020, Vol 12(1), Article 1770028.
- Dang X et al, “Clinical Investigation of Large Volume Subcutaneous Delivery up to 25 mL for Lean and Non-Lean Subjects”. Pharm Res, 2024, Vol 41(4), pp 751–763.
- Allmendinger A et al, “Rheological characterization and injection forces of concentrated protein formulations: an alternative predictive model for non-Newtonian solutions”. Eur J Pharm Biopharm, 2014, Vol 87(2), pp 318–328.
- Mazaheri M et al, “Monitoring of Visible Particles in Parenteral Products by Manual Visual Inspection-Reassessing Size Threshold and Other Particle Characteristics that Define Particle Visibility”. J Pharm Sci, 2024, Vol 113(3), pp 616–624.