To Issue 159
Citation: Janssen P, “Change is in the Air: the Future Climate of Inhaled Medication”. ONdrugDelivery, Issue 159 (Apr/May 2024), pp 34–39.
Pauline Janssen, discusses the various available devices for pulmonary delivery of medications, with a deeper dive into the challenges presented by replacing pressurised metred dose inhaler propellants with high global warming potential with new alternatives, or whether the optimal solution could be phasing out pressurised metered dose inhalers in favour of dry powder inhalers.
INTRODUCTION
Breathing: something that comes naturally but is vital to our existence. The lungs are amongst the most important human organs and are continuously in contact with our environment via the exchange of air. The lungs are therefore an entrance portal for pathogens and viruses, potentially leading to respiratory diseases, such as covid-19.1 Other common respiratory diseases include asthma and chronic obstructive pulmonary disease (COPD). People suffering from these diseases experience problems with breathing and obstructed airflow. Hence, pulmonary treatment is required.
Pulmonary delivery is a non-invasive, patient-friendly route of drug administration that offers several advantages over other delivery routes. Pulmonary delivery avoids the first-pass effect of the gastrointestinal (GI) tract and liver, which results in high bioavailability.2 The lungs are reported to be more permeable to small-molecule and macromolecule drugs than any other portal of entry into the body,3 which means that lower doses are required to reach the target effective dose, reducing the systemic side effects at off-target locations in the body.
Another advantage of pulmonary delivery relates to the rapid and predictable onset of action associated with it. This is due to the large surface area of the lungs available for absorption, even though the lungs compromise only a relatively low mass fraction of the body.1
“An advantage of breath-actuated pulmonary delivery is that only limited levels of patient co-ordination are required.”
Many different inhalation devices are available in the market to enable pulmonary drug delivery. These devices differ in their efficiency, internal resistance, formulation of medication, particle size, velocity of the aerosol plume and ease of use.6–8 These devices can be categorised into four main types – dry powder inhalers (DPIs), nebulisers, soft mist inhalers (SMIs) and pressurised metered dose inhalers (pMDIs).9 Each type of device has its own advantages and disadvantages.
TYPES OF RESPIRATORY DEVICE
Dry Powder Inhalers
DPIs are compact and portable devices that are designed to deliver medication in the form of a dry powder, which can be beneficial for the physical and chemical stability of a formulation. Generally, a single quick inhalation event is sufficient to deliver a complete dose to the lungs. Doses are typically in the microgram range, but particle engineering technologies can allow expansion to milligram ranges. A patient breathing through the device actuates the dispersion of the formulation into the inhaled air. The energy from the patient’s breath therefore needs to be sufficient to disperse the particles into the air stream. An advantage of breath-actuated pulmonary delivery is that only limited levels of patient co-ordination are required.
DPIs are a relatively new type of pulmonary delivery device, entering the respiratory market in 1967.10 Currently, about 22% of all inhalation devices sold worldwide are DPIs and their market share is expected to increase in the coming years.11
Nebulisers
A nebuliser is a type of inhalation device used to administer medication in the form of a mist. The drug is dissolved or suspended in a polar solvent, which is turned into an aerosol by external energy. As a result, nebulisers are typically bulky, noisy devices that require an external power source. Jet nebulisers, for example, create a mist by flowing compressed air or oxygen at a high velocity over the liquid, while ultrasonic nebulisers create a mist via a high-frequency ultrasonic wave.
The mist created by a nebuliser is inhaled for a prolonged period of approximately 20 minutes through a mouthpiece or mask.2 Drug waste levels are relatively high, requiring larger doses for administration. The main advantage of nebulisers is the absence of a need for strong breathing or co-ordination, allowing patients unable to carry out active inhalation to use these devices.12
Nebulisers are mainly used for incidental or temporary administration by healthcare providers. Consequently, nebulisers account for only a relatively small share of the respiratory market (9%) and the amount of consumed units grew by less than 1% in the 2018–2022 period.11
“SMIs are relatively new in the market, resulting in limited availability and typically higher costs than more established inhaler types.”
Soft Mist Inhalers
SMIs contain liquid formulations similar to those in nebulisers. However, SMIs are small, portable, handheld inhalers that do not require a power supply. Drug deposition is not actuated by the patient’s breath, but rather a variety of principles that are used to create a slow-moving, long-sustaining aerosol cloud upon actuation.13 The advantage of this aerosol cloud is that the co-ordination between actuation and inspiration is less critical than for pMDIs, although a long breath is required for optimal inhalation. SMIs can deliver doses in the microgram range, typically with a high lung deposition that is less dependent on the inspiratory flow of the patient.14 SMIs need to be primed before the first use, which needs to be partly repeated if a device has not been used for more than three days.15
SMIs are relatively new in the market, resulting in limited availability and typically higher costs than more established inhaler types.16 Currently, their market share is 3% with a growth of 6% in consumed units over the 2019–2022 period.
Pressurised Metered Dose Inhalers
pMDIs are portable, easy-to-use devices for the administration of medication via a short burst of aerosolised medicine. pMDI formulations contain drug particles that are dissolved or dispersed in a liquified propellant. Drug deposition via the nozzle is activated by pressing down the top of the canister. Consequently, expansion of the propellant results in atomisation of the formulation into small droplets. Actuation of a pMDI should be done in parallel with inhalation by the patient to administer the medication effectively. Besides the difficult co-ordination of actuation and inhalation, patients have also indicated that it is difficult to determine when their pMDI is empty.12
The pMDI was the first portable inhalation device to be launched in the market, starting with the introduction of the first pMDI in 1956.17 Since then, pMDIs have become the most widely prescribed inhalation device for drug delivery to the respiratory tract.12 This is mainly driven by the relatively low cost of pMDIs, and therefore the wide availability of this type of device. The pMDI segment accounts for 65% of the global respiratory market, with a moderate growth of 1.8% in consumed units since 2018.11
The main challenge of pMDIs is the carbon footprint associated with them, which is playing a pivotal role in the expected growth of this inhaler type. Currently used propellants have a substantial environmental impact, and reformulating to available alternatives comes with challenges.
“While the ozone-friendly HFAs developed to replace CFCs do not deplete the ozone layer, they are potent greenhouse gases with global warming potential (GWP) ranging from 1300 2900g CO2 equivalents.”
THE ENVIRONMENTAL CHALLENGE OF pMDI PROPELLANTS
While efficacy and safety have always been top priority in the selection of a medication, increased attention is now being paid to the environmental impact as well. In particular, pMDIs are under scrutiny due to their propellants being potent greenhouse gases. The propellant comprises the bulk of any pMDI formulation. It is required to be toxicologically safe, non-flammable and chemically inert, with appropriate boiling points and densities, as well as needing to provide the same vapour pressure regardless of whether the pMDI canister is full or empty.18 Initially, pMDIs were formulated with chlorofluorocarbons (CFCs) as propellants. However, in 1987, the Montreal Protocol was signed to control and phase out ozone-depleting substances, including CFCs. CFC-propelled pMDIs have been the only major exemption from the protocol under a clause for “essential use”, but only for a limited period of time while no alternatives were available.19
Hydrofluoroalkanes (HFAs) have been found to not deplete stratospheric ozone and are proven to be safe as pharmaceutical excipients.20 Thus, HFA 227 and HFA 134a were developed to replace CFC propellants in pMDI formulations. These HFAs could not directly substitute for CFC propellants, as previously used excipients and hardware components were not compatible with HFA formulations, due to the different physiochemical properties. Therefore, significant effort and investments were required to develop new device hardware and formulation approaches.18 It has taken a couple of decades to complete, but transition has been a worldwide collaborative success.
While the ozone-friendly HFAs developed to replace CFCs do not deplete the ozone layer, they are potent greenhouse gases with global warming potential (GWP) ranging from 1300–2900 g CO2 equivalents.21 So, even though large improvements have been achieved in the environmental impact of pMDIs by switching from CFCs to HFAs, HFAs are also expected to have a substantial impact on global warming if production is not controlled.22 Phasing out of HFA 134a and HFA 227 is now planned under the Kigali Amendment to the Montreal Protocol in 2016,23 as well as through national F-gas regulations in Europe24 and the US.25 Figure 1 shows an overview of the GWP of the different propellants. Two propellants have been proposed for pMDIs with significantly lower GWPs, those being HFA 152a and HFO 1234ze(E).
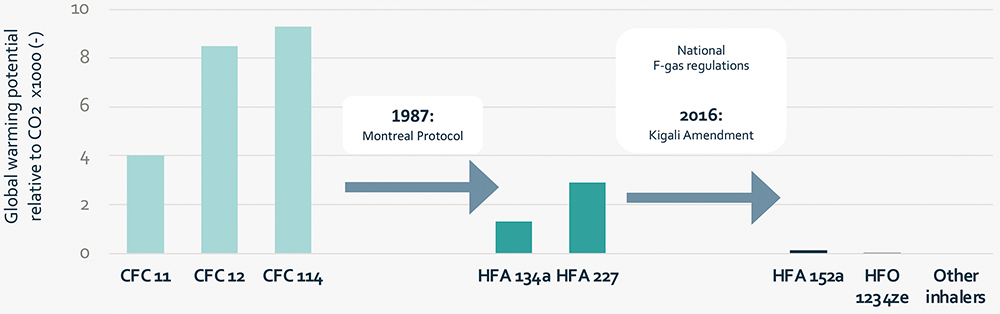
Figure 1: Global warming potential (relative to CO2) of different fluorinated aerosol propellants.18,26
Alternative Propellants
So, what is the future for pMDIs? Is there even still potential for them? It must be noted here that no consensus exists on what is the best inhaler for patients, and the most appropriate inhaler can only be identified on a case-by-case basis. In the majority of cases, switching patients from pMDI medication to DPI medication results in improved or equal disease control.27–29 For certain groups of patients, however, an impaired ability to inhale quickly could hinder the correct use of a DPI.26 With this in mind, it will be impossible to fully replace pMDIs, as they are required from a clinical perspective for reliever medication at the least.
To reduce the environmental impact of critical pMDIs, there is a need for low-GWP propellants to replace those currently in use. Many pharmaceutical companies, however, have spent a lot of effort and money to support the phase-out of CFCs and are reluctant to repeat this process. Two propellants have been proposed for pMDIs with significantly lower GWP – HFA 152a and HFO 1234ze(E). One of the critical activities for the introduction of these propellants is the generation of long-term human safety data that may be required before getting market authorisation. Furthermore, both HFA 152a and HFO 1234ze(E) have some patent applications relating to its use as a medical propellant, making implementation of these propellants more complex.30–34
One of the challenges associated with HFA 152a in particular is that it is a flammable propellant, with an explosive limit of 3.9% by volume in air at room temperature.35 Safe manufacturing processes for HFA 152a still need to be developed, which will necessitate large investments for completely new infrastructures for the production of low-GWP pMDIs.
HFO 1234ze(E) is the most attractive propellant from an environmental perspective. Additionally, as Table 1 indicates, this propellant is more similar to HFA 134a and HFA 227 in relevant physical properties, indicating that there are likely to be fewer challenges with reformulation. The main challenge associated with HFO 1234ze(E), however, is that it is a newer propellant with a more complex chemical synthesis, resulting in higher production costs. This will likely lead to a higher supply price compared with other propellants, putting the costs of the final pMDI under pressure. As cost effectiveness has been the main advantage of pMDIs, it might be difficult to make a convincing business case for the implementation of HFO 1234ze(E).
Propellant | CFC 11 | CFC 12 | CFC 114 | HFA 134a | HFA 227 | HFA 152a | HFO 234ze(E) |
Chemical Formula | CCl2F | CCl2F2 | C2ClF4 | C2F4H2 | C3F7H | C2F2H4 | C3F4H2 |
GWP (CO2 equivalent) | 4,000 | 8,500 | 9,300 | 1,300 | 2,900 | 138 | <1 |
Liquid Density at 20°C (g/mL) |
1.49 | 1.3 | 1.47 | 1.21 | 1.41 | 0.91 | 1.29 |
Dipole moment (debye) | 0.46 | 0.51 | 0.50 | 2.06 | 0.93 | 2.26 | 1.44 |
Water solubility at 25–30°C (ppm) |
130 | 120 | 110 | 2220 | 610 | 2200 | 225 |
Boiling point (°C) | 22.8 | -29.8 | 3.6 | -25.8 | -17.3 | -24.7 | -18.9 |
Table 1 Physiochemical properties and the GWP (relative to CO2) of different fluorinated aerosol propellants.18
“Studies have shown that switching from a pMDI to a DPI medication typically does not affect the disease control for patients.”
DPIs AS AN ALTERNATIVE FOR MDIs
The price of more environmentally friendly propellants is increasing the cost of pMDIs. With challenges in patient adherence, it remains a question if switching to low-GWP pMDIs is the right way forward. There is presently an opportunity to switch to different types of inhalers that are equivalent in cost and more environmentally friendly. Figure 2 shows the GWP over the lifecycle of different types of inhalers, indicating large differences between the different types of pMDIs, due to the amount and type of propellants used. In general, pMDIs with HFA propellants have about 30 times the GWP of equivalent DPIs. Switching to DPIs would therefore provide a huge opportunity to reduce greenhouse gas emissions.
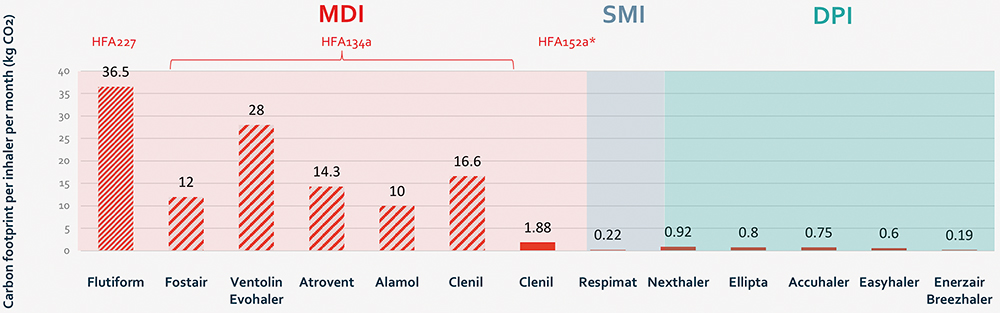
Figure 2: Graph showing the indicative monthly carbon footprint in lifecycle analyses. *Clenil HFA 152a shows the predicted carbon footprint of a potential future HFA 152a-containing pMDI.28
Research has been performed to evaluate if transferring patients from pMDIs to DPIs would result in a change in disease control. For example, Singh et al compared the performance of DPI and pMDI formulations of beclomethasone/formoterol fumarate. The study concluded that the performance of an extrafine Fostair® 100/6 μg NEXThaler (Chiesi) DPI was comparable to an extrafine Foster® 100/6 μg pMDI when administered as reliever therapy after methacholine induced bronchoconstriction to mimic an asthma attack.36 The degree of bronchodilation achieved with the DPI and pMDI was practically identical, both in magnitude and onset of action. The contribution of these two devices (based upon 120 doses) over the entire lifecycle of the device was calculated by Panigone et al. A Fostair® 100/6 μg pMDI was calculated to have a GWP of 94.4 g CO2 equivalents per actuation, while the Fostair® 100/6 μg DPI was calculated to have a GWP of 7.63 g CO2 equivalents per actuation.37
Studies have shown that switching from a pMDI to a DPI medication typically does not affect the disease control for patients. Woodcock et al studied patients with symptomatic asthma who switched from pMDI medication to the Ellipta DPI (GSK). By switching to a DPI, patients more than halved their inhaler’s carbon footprint, without any loss in asthma control.27 Conversely, a worsening of asthma control was observed in the UK when patients were switched from a DPI to a pMDI for financial reasons.28
Interchangeability of different device types was also confirmed by the distribution of inhaler sales across different countries in Europe, as indicated in Figure 3. In the UK, approximately 70% of the total retail units sold were related to pMDI treatment, while in Sweden the number was only 10%. Differences were not expected to be the result of different health indications per country,38 indicating that different device types can be used effectively for treatment of the same disease.
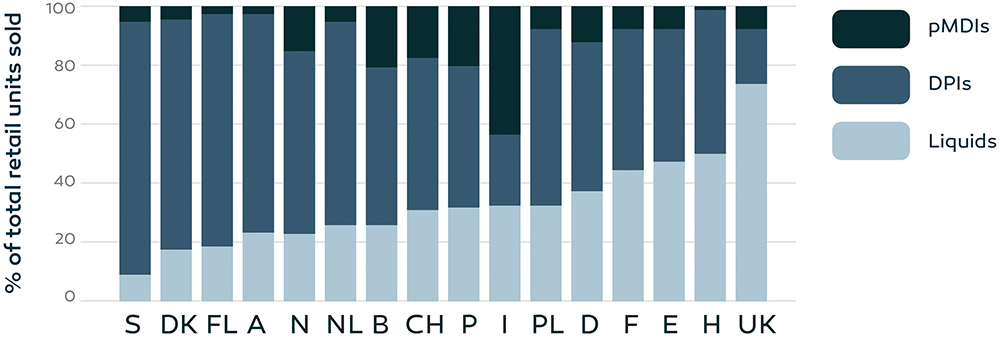
Figure 3: Retail sales of inhalation devices, expressed as percentages of the total sales, in 16 European countries over the time period 2002–2008 (A, Austria;
B, Belgium; CH, Switzerland; DK, Denmark; E, Spain; F, France; FL, Finland; H, Hungary; I, Italy; D, Germany; N, Norway; NL, Netherlands; P, Portugal; PL, Poland; S, Sweden; UK, United Kingdom).38
CONCLUSION
Pulmonary drug delivery is a non-invasive, patient-friendly route of administration that is getting increased interest due to the advantages it has to offer. Different types of inhalers exist, and the most appropriate inhaler can only be identified on a case-by-case basis. While efficacy and safety have always been a top priority in choosing medication, there is now increased attention being paid to the environmental impact as well.
The global warming debate has already been a challenge for the pMDI industry for decades. A significant amount of effort and money has already been spent to replace CFC propellants with more environmentally friendly alternatives. Two strategies that have been proposed to reduce the GWP of pMDIs include switching to low-GWP propellants and switching to other types of device when possible. Two propellants have been proposed for the development of pMDIs with significantly lower GWP, each with their own challenges and associated costs. HFA 152a is a flammable propellant, which means that safe manufacturing processes will need to be developed, requiring large investments for completely new infrastructure. HFO 1234ze(E) is less flammable and is the most attractive propellant from an environmental perspective, but it is a newer propellant with a more complex chemical synthesis, resulting in higher production costs. As prices of currently used propellants and future propellants increase the costs of pMDIs, the main advantage of pMDIs over other devices is diminishing. Multiple studies have shown that DPIs typically result in equivalent or better disease control than pMDIs. Switching therapies from pMDIs to DPIs could therefore present a major opportunity to reduce greenhouse gas emissions, without reducing disease control.
REFERENCES
- Anderson S et al, “Inhaled Medicines: Past, Present, and Future”. Pharmacol Rev, 2022, Vol 74(1), pp 48–118.
- Cheng Y et al, “Compatible Stability and Aerosol Characteristics of Atrovent® (Ipratropium Bromide) Mixed with Salbutamol Sulfate, Terbutaline Sulfate, Budesonide, and Acetylcysteine”. Phamaceutics, 2020, Vol 12(8), Article 776.
- Patton JS, Fishburn CS, Weers JG, “The lungs as a portal of entry for systemic drug delivery”. Proc Am Thorac Soc, 2004, Vol 1(4), pp 388–344.
- Ibrahim M, Verma R, Garcia-Contreras L, “Inhalation drug delivery devices: technology update”. Med Devices (Auckl), 2015, Vol 8, pp 131–139.
- Kim ES, Plosker GL, “AFREZZA® (insulin human) Inhalation Powder: A Review in Diabetes Mellitus”. Drugs, 2015, Vol 75(14), pp 1679–1686.
- Laube BL et al, “What the pulmonary specialist should know about the new inhalation therapies”. Eur Respir J, 2011, Vol 37(6), pp 1308–1331.
- Dolovich MB, “Device selection and outcomes of aerosol therapy: Evidence-based guidelines: American College of Chest Physicians/American College of Asthma, Allergy, and Immunology”. Chest, 2005, Vol 127(1), pp 335–371.
- Dolovich MB, Dhand R, “Aerosol drug delivery: developments in device design and clinical use”. Lancet, 2011, Vol 377(9770), pp 1032–1045.
- Doyle GR, McCutcheon JA, “6.6 Administering Inhaled Medications” in “Clinical Procedures for Safer Patient Care”. BCcampus, 2015.
- de Boer AH, Hagedoorn P, Grasmeijer F, “Dry powder inhalation, part 2: the present and future”. Expert Opin Drug Deliv, 2022, Vol 19(9), pp 1045–1059.
- IQVIA data, 2022.
- Lavorini F, Fontana GA, Usmani OS, “New inhaler devices – the good, the bad and the ugly”. Respiration, 2014, Vol 88(1), pp 3–15.
- Lavorini F, “The challenge of delivering therapeutic aerosols to asthma patients”. ISRN Allergy, 2013, Vol 2013, Article 102418.
- Anderson P, “Use of Respimat Soft Mist inhaler in COPD patients”. Int J Chron Obstruct Pulmon Dis, Vol 1(3), pp 251–259.
- Gerald LB, Dhand R, “Patient education: Inhaler techniques in adults (Beyond the Basics)”. UpToDate, Dec 2022.
- Hodder R, Price D, “Patient preferences for inhaler devices in chronic obstructive pulmonary disease: experience with Respimat Soft Mist inhaler”. Int J Obstruct Pulmon Dis, 2009, pp 381–390.
- Preedy EC, Prokopovich P, “2 – History of inhaler devices” in “Inhaler Devices: Fundamentals, Design and Drug Delivery” (Prokopovich P ed). Woodhead Publishing, 2013.
- Myrdal PB, Sheth P, Stein SW, “Advances in metered dose inhaler technology: formulation development”. AAPS PharmSciTech, 2014, Vol 15(2), pp 434–455.
- Woodcock A, “The president speaks: prevention is best: lessons from protecting the ozone layer”. Thorax, 2012, Vol 67(12) pp 1028–1031.
- Hendeles L, Colice GL, Meyer RJ, “Withdrawal of albuterol inhalers containing chlorofluorocarbon propellants”. N Engl J Med, 356(13), pp 1344–1351.
- Wilkinson AJK et al, “Costs of switching to low global warming potential inhalers. An economic and carbon footprint analysis of NHS prescription data in England”. BMJ Open, 2019, Vol 9(10), Article e028763.
- Velders GJM et al, “The importanceof the Montreal Protocol in protecting climate”. Proc Natl Acad Sci USA, 2007, Vol 104(12), pp 4814–4819.
- “About Montreal Protocol”. Web Page, UN Environment Programme, Accessed Mar 2024.
- “Regulation (EU) No 517/2014 of the European Parliament and of the Council of 16 April 2014 on fluorinated greenhouse gases and repealing Regulation (EC) No 842/2006”. European Commission, 2014.
- “F-Gas Partnership Programs”. US Environmental Protection Agency, Web Page, Accessed Mar 2024.
- Pritchard JN, “The Climate is Changing for Metered-Dose Inhalers and Action is Needed”. Drug Des Devel Ther, 2020, Vol 14, pp 3043–3055.
- Woodcock A et al, “Effects of switching from a metered dose inhaler to a dry powder inhaler on climate emissions and asthma control: post-hoc analysis”. Thorax, 2022, Vol 77(12), pp 1187–1192.
- Wilkinson A, Woodcock A, “The environmental impact of inhalers for asthma: A green challenge and a golden opportunity”. Br J Clin Pharmacol, 2022 Vol 88(7), pp 3016–3022.
- Janson C et al, “Carbon footprint impact of the choice of inhalers for asthma and COPD”. Thorax, 2020, Vol 75(1), pp 82–84.
- Knopeck GM et al, “Medicament delivery formulations, devices and methods”. Patent US20160324778A1, 2016.
- Pham HT, Singh RR, “Sprayable composition comprising a propellant composition comprising 1,3,3,3-tetrafluoropropene (HFO-1234ze) and a material to be sprayed”. Patent ES2551936T5, 2015.
- Corr S, Noakes TJ, “Stable pharmaceutical compositions comprising indacaterol and 1,1-difluoroethane (hfa-152a) suitable for use in metered dose inhalers (mdis)”. Patent CA3037080C, 2018.
- Corr S, Noakes TJ, “Stable pharmaceutical compositions comprising salmeterol and 1,1-difluoroethane (hfa-152a) suitable for use in metered dose inhalers (mdis)”. Patent CA3037107C, 2018.
- Corr S, Noakes TJ, “Stable pharmaceutical compositions comprising glycopyrrolate and 1,1-difluoroethane (hfa-152a) suitable for use in metered dose inhalers (mdis)”. Patent CA3037092C, 2018.
- “HP 152a Aerosol Propellant”. Chemours, 2017.
- Singh D et al, “Comparison of the effect of beclometasone/formoterol in asthma patients after methacholine-induced bronchoconstriction: A noninferiority study using metered dose vs. dry powder inhaler”. Br J Clin Pharmacol, 2019, Vol 85(4), pp 729–736.
- Panigone S et al, “Environmental impact of inhalers for respiratory diseases: decreasing the carbon footprint while preserving patient-tailored treatment”. GMJ Open Respir Res, 2020, Vol 7(1), Article e000571.
- Lavorini F et al, “Retail sales of inhalation devices in European countries: so much for a global policy”. Respir Med, 2011, Vol 105(7) pp 1099–1103.