To Issue 146
Citation: Davies W, “Designing Sustainability into Pharmaceutical Devices”. ONdrugDelivery, Issue 146 (Apr/May 2023), pp 6–11.
Will Davies discusses how to address the challenges of designing sustainability into pharmaceutical devices.
“It is self-evidently in the interests of all industry stakeholders to maintain a viable and responsible industry.”
We are all aware of the climate crisis. With governments recently battling over national responsibilities at COP27, we all need to look at our own behaviours, habits and practices – and this extends from an individual level all the way through to global industrial complexes. As a whole, the healthcare industry’s climate footprint, at 2.2 gigatonnes carbon dioxide equivalent (CO2e), is equivalent to 4.4% of net global emissions – if it were a country, it would be the fifth largest in the world.1
Given that emissions and healthcare outcomes are closely linked, this creates a deep irony for the industry. Traditionally (and justifiably), so much emphasis has been given to safety, hygiene and quality that virgin materials, single-use devices and cautious packaging have been prioritised over any fledgling sustainability concerns. The standard pharmaceutical model has been “formulate – fill – distribute – deliver – repeat”; a single-use pathway that is typically material and emission heavy. Now, with tangible effects of global warming being felt around the world, and major healthcare bodies such as the NHS in the UK declaring that it will no longer purchase from suppliers that do not meet its commitment to net zero by the end of the decade,2 the pressure is on the healthcare industry to reform its practices and standard models in order to significantly reduce its footprint, all the way down to net zero.
The NHS’s landmark report, “Designing a net zero roadmap for healthcare” (intended to be globally influential), identifies that more than six megatonnes CO2e can be saved from the NHS England footprint through the reduction of single-use plastics, device reuse, process and product innovation, and pharmaceutical suppliers meeting the NHS’s net-zero commitments. These are all factors that the medical and pharmaceutical supply chain has a direct influence on. This equates to 37% of the total NHS footprint of 16.5 Mt CO2e; if that same 37% reduction can be applied globally, that’s an emissions reduction of 814 Mt CO2e – an enormous figure.
On top of customer specification for reduced footprint comes increased legislation, not least in the form of extended producer responsibility.3 Originating in the EU but steadily rolling out across US states and elsewhere, this places a responsibility for post-consumer waste back onto the original manufacturer. Although not yet extended to the pharmaceutical industry, it is increasingly embracing consumer electronics, which includes the wellness and digital health industries, and it is not hard to imagine it moving further into the medical sector in the future.
“One of the key changes required is to transition to a product-as-a-service model.”
ADDRESSING THE CHALLENGE
So how do we do it? How do we modify/reinvent/disrupt the well-established standard models of the behemoth pharmaceutical industry to slash these emissions? It is self-evidently in the interests of all industry stakeholders to maintain a viable and responsible industry. Those involved in the design and development of drug delivery devices and system designers are ideally placed to address those key areas identified by the NHS: single-use plastics, reusable devices, and process and product innovation.
We see the word “sustainable” used extensively these days, particularly in corporate literature and advertising as manufacturers aim to demonstrate their corporate social responsibility credentials when describing new product ranges in comparison with older versions, and frequently used alongside the phrase “minimising our carbon footprint”. But is it really sustainable – and are they really minimising their footprint?
Take, for example, a new hypothetical range of packaging – single material, 100% recyclable, 50% lighter and smaller than the previous range, etc. Great effort, and a significant reduction in emissions related to manufacture and transport. But, to be truly sustainable, it must be 100% recycled at end of life, otherwise it is just a continued – albeit reduced – consumption of a finite resource, which by definition is not sustainable. There are various definitions of sustainability, but it is broadly agreed to refer to the ability of maintaining something at a certain rate or level. Therefore, if a practice is depleting a resource at any rate, it is not sustainable. To guarantee 100% recycling rates and true sustainability, the manufacturer must take responsibility for the end-of-life materials and return them to the supply chain within a closed loop; otherwise they are relying on existing recycling streams, which are full of holes (not least the consumer – less than 50% of plastic packaging is recycled globally on average).4 Therefore, the claim that they are minimising their footprint is only true within the confines of the existing business model – to minimise it absolutely would be to change that model to one that closes the loop.
CLOSING THE LOOP
It is widely recognised that the primary – perhaps only – route to true sustainability is to adopt a circular economy model. Popularised by Braungart and McDonough in Cradle to Cradle (2002), a leading contemporary exponent of this – and possibly provider of the clearest and most compelling information – is the Ellen MacArthur Foundation. In summary, this is a model that takes the principles of the three Rs (reduce, reuse, recycle) to its logical extreme. It differentiates between renewable and finite material streams and establishes loops to return these materials either to nature or into new products indefinitely, minimising waste and negative external effects (Figure 1). This, therefore, is the ultimate method by which those key NHS-derived criteria are satisfied: elimination of single-use plastics by returning and recycling such materials; the development of reusable devices through their return and remanufacture; and ongoing process and product innovation resulting in the minimising of resource use in the first place.
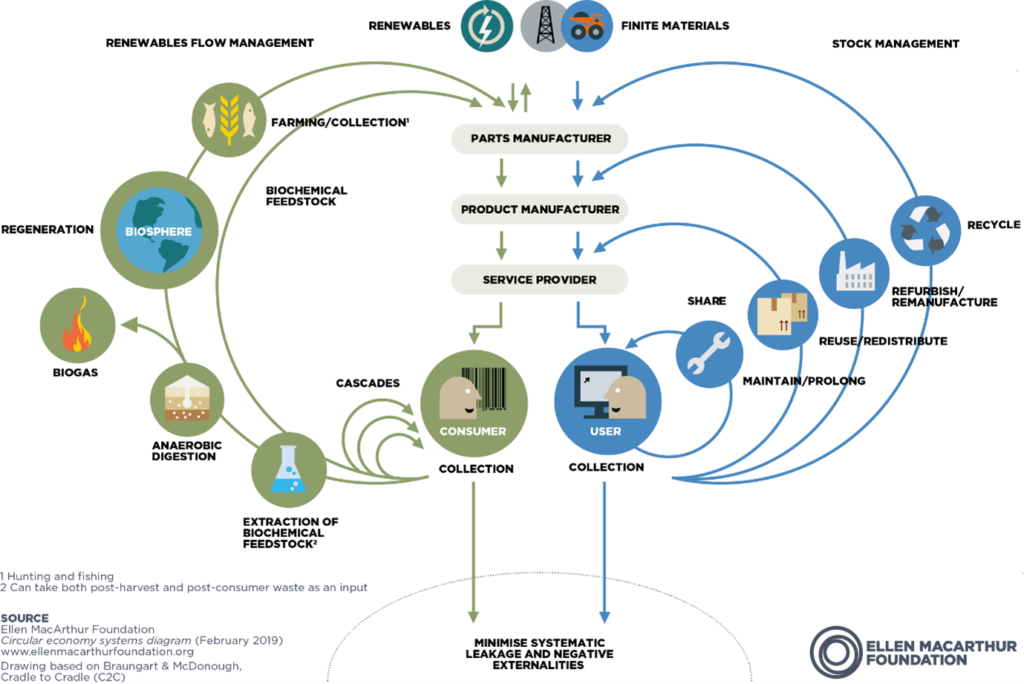
Figure 1: Circular economy butterfly diagram.
All very well in theory. But how does that translate into practice? One of the key changes required is to transition to a product-as-a-service model, whereby the function of the device (drug delivery, diagnosis, monitoring, etc) is the commodity, and the device itself is merely a vehicle for that commodity and remains the property and responsibility of the supplier. Philips is one of the pioneers of this approach; initially with large items, such as MRI and CT scanners, which it would take back for reconditioning, and now increasingly with smaller items of equipment, such as monitors and ventilators.
One key advantage of this system is that it also allows the equipment to be upgraded as it is reconditioned, perhaps with just software changes or limited component changes, but either way without the virgin material demands and associated emissions of a completely new product; even though the reconditioned item appears new (or as good as) to the next user, at lower cost. At inception of this strategy in 2012, Philips Chief Executive Officer Frans van Houten was so confident that it would increase competitiveness, deliver cost savings and form stronger customer relationships that he implemented some key performance indicators specifically focused on circularity and sustainability, with a commitment to 25% of revenue from circularity by 2025.5
Very laudable and compelling. But CT scanners and hospital monitors are a very different proposition from autoinjectors, wearable devices and blood glucose monitors, which are patient orientated. These kinds of devices present several challenges to circularity, the main one being user behaviour. Whereas a device such as a ventilator sits within a controlled environment (a hospital) and is managed by trained users under strict policies, making it easy to track and recover, instead we have a very large number of small devices that are taken into uncontrolled environments (the home), with no monitoring and no incentive or mechanism to return the device at the end of its life. At present, such devices tend to be built with a focus on low cost, rapid assembly and robustness; all of which make disassembly difficult. What’s more, a used device may well present significant biohazards, especially those with needles.
“It is unlikely that devices currently on the market and designed for today’s single-use model can be efficiently incorporated into a circular economy model.”
ENTER DESIGN
The User Challenge
The first challenge is to address consumer behaviour and encourage (or compel) the user to return the device. Novo Nordisk is trialling an autoinjector return programme6 in Denmark, whereby the user takes their old injectors to the pharmacy, from where they are shipped to a reprocessing facility. As it happens, they are not currently turned into new autoinjectors, but instead downcycled into other products, so the loop is not fully closed – still, much better than landfill. GSK ran a similar scheme in the UK, called “Complete the Cycle”, to collect used inhalers but – after a reported return rate of just 0.4% (which is still two million inhalers) over 10 years – discontinued it in 2020.7
There is a key barrier here: asking the user to take their devices to a collection point, such as a pharmacy. This requires the user to be sufficiently motivated and organised, and also to travel. The travel element itself is particularly problematic, as this feeds into the “last-mile” factor of product-related emissions, especially if a private internal combustion engine8 vehicle is used, by increasing the emissions associated with the device’s lifecycle. Similarly, travelling to a pharmacy to collect a new device in the first place is also a contributory factor to the product’s emissions.
So, can good design promote increased user engagement and facilitate a worthwhile return rate? Financial deposit schemes – akin to bottle return deposits – are not reliable and run counter to the ethos of health services such as the NHS where “free at point of use” is sacrosanct. User education alone is optimistic, relying on goodwill and motivation. The key is to keep the user engaged whilst removing any burden or sense of chore.
Let us use autoinjectors in a hypothetical example of how it might work. A patient gets a new or repeat prescription of a course of injections from their healthcare professional (HCP). Rather than the standard model of the patient collecting the prescription from the pharmacy, they instead receive a package direct to their door via a trackable courier service from the manufacturer – this would be arranged by the HCP or pharmacist via a secure online portal system. The package containing the autoinjectors could be a letterbox friendly chocolate box format – a slim rectangular cardboard box, fully recyclable and attractively printed, containing a recyclable pulp tray in which is nestled the course of autoinjectors. A bespoke label for the prescription is adhered to the tray, identifying the application date for each injector (Figure 2).
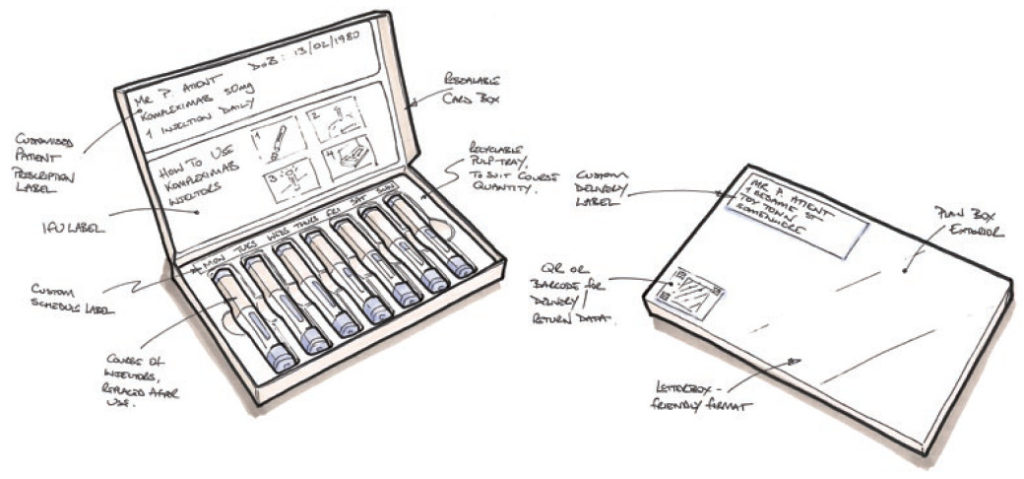
Figure 2: Sketch of letterbox-friendly autoinjector packaging suggestion.
Once the injection is complete, the user simply puts the injector back in its original position in the tray. When all injectors have been replaced, the user reseals the box and it is ready for collection for return and reprocessing by the same courier service – automatically scheduled according to the prescription and dosage rate. Should the course need to continue beyond the number of injectors in one box, then subsequent deliveries would also be pre-scheduled.
The entire process is managed automatically, reducing margin for error, and no extra effort is required on the part of the patient – injection reminders, delivery information and so on could even be sent to the patient’s phone. The use of a courier, dedicated to delivering and collecting multiple prescriptions and devices (such delivery services are already commonplace) vastly reduces those last-mile emissions whilst using specialists in handling medication and maintaining any necessary environmental conditions. The box would provide a large amount of space for print and graphics – keeping the user informed and engaged with the process.
Thus, medication is provided as a service rather than a product, with the device itself only temporarily relinquished, and the burden of effort on the user greatly diminished. A huge additional benefit of this system would likely be a significant increase in adherence and compliance – one of the healthcare industry’s other major challenges – due to the use of automatic scheduling and dose reminders.
The Return Challenge
So, our box of used devices has been picked up and is making its way back to close the loop. But back to where? The devices may be comprised of a mix of high-specification plastics, glass, metals and electronics, now with some biohazard thrown in. Do they go to the original manufacturing plant perhaps, which has a disassembly and remanufacturing facility? This could work efficiently if well set up, although it might present logistical problems as it is likely to be a single plant, which is drip-fed devices from global sales regions. Perhaps better could be regional disassembly hubs – probably operated by third parties dealing with other products as well – that receive the devices and separate them into various controlled streams. These streams would likely be:
a. Reusable components or modules, subsequently returned to the assembly plant for integration into remanufactured devices.
b. Damaged, soiled or contaminated components to be cleaned and ground into recyclate and returned to the original component supplier for reprocessing.
c. Organic materials, such as card packaging or pulp trays, recycled into new paper/card stock or anaerobically digested to create biogas for power generation (for example).
As these three streams seem likely to head off to different destinations, this favours the regional disassembly hub model. Each stream would be shipped in bulk when sufficient volumes are reached, minimising emissions.
Stream A
How do we know if a component or module in stream A is fit for reuse without laborious and expensive manual inspection? Some manual intervention will, of course, always be required, although there are sophisticated machine vision systems10 available now that would take some of the burden out of this task, for instance recognising and rejecting damaged components. Parts or modules could be tested for function at a stage on the reassembly line prior to integration. However, there are now a number of companies developing component labelling systems that will enable rapid identification and lifecycle tracking, facilitating automatic rejection of components that are nearing their lifetime number of actuations. An example of a company currently focusing on packaging is Polytag (Deeside, Wales).
Stream B
Can material recycling for stream B satisfy the quality demands for medical devices? With current recycling processes, this is unlikely – but current processes are focused on the mass recycling of packaging and similar consumer waste in just a few broad streams, resulting in contamination and ultimately material downcycling. However, with an increased focus on circularity and enhanced collaboration between the manufacturing and recycling industries – particularly with advanced component identification – it is entirely foreseeable that segregation and recycling processes could be refined to cope with and preserve a range of engineering polymers and metal alloys, for reintegration into the original manufacturing process. Designers and engineers would initially have to be careful to select materials that can be recycled indefinitely without degradation (such as polypropene (PP)) to facilitate this, just as raw-material suppliers will need to increase the development of fully recyclable (zero-degradation) polymers to meet engineering demand.
The Device Design Challenge
It is unlikely that devices currently on the market and designed for today’s single-use model can be efficiently incorporated into a circular economy model – some elements may be recovered and reused effectively but a device that has not been designed for disassembly will probably throw up multiple barriers. One-way fastening features (snap fits), adhesives and overmoulds could all be such examples. Therefore, to fully embrace circularity, devices will need to be redesigned accordingly. This redesign work could address the following areas:
a. Labels: useful not only for displaying instructions, graphics and logos, labels can also be used on a device to hide features such as screws and snaps. Typically, product labels are made from polyethylene terephthalate (PET), PP or similar and are difficult to remove, often leaving behind a contaminating adhesive residue. However, there are now solutions, such as dissolvable label products, that would overcome this – the device or component simply needs to be placed in a bath for a short while. An example of a company developing dissolvable labels is SmartSolve (OH, US). For devices where exposure to water is not an option, labels could be readily peelable with a dedicated releasable adhesive (perhaps with a controlled method of accessing a peel start point).
b. Reuse or Recycle: some components or sub-assemblies of a product will remain fully functional and in as-new condition post use, for instance sprung drive systems or printed circuit board (PCB) assemblies. These could be designed as single recoverable units, perhaps requiring a reset procedure but otherwise ready to slot straight into a new assembly. Some may require a decontamination procedure, which could be achieved in – for example – a chlorine solution (perhaps in combination with dissolving a label), an ultrasonic bath or with ultraviolet light. Conversely, some components will be soiled, damaged or worn to the extent that they cannot be reused and should be recycled instead. This would likely include product casings and interaction points such as handles or buttons – and, of course, needles and vials. These should ideally be comprised of materials that can readily be recycled back into the original components without degradation, but otherwise could be downcycled into alternative products.
c. Design for Disassembly: this must be embedded into the design process to the same degree that design for assembly and design for manufacture are. Quality standard BS-EN/ISO 8887-1:2006 (currently being updated) provides guidance in this area; however, there are some fundamental concepts that can be pursued to facilitate efficient disassembly. Firstly, just as jigs and fixtures for assembly are often designed in parallel with the device design, so too should disassembly jigs and fixtures. These could be used to release snap features, neutralise springs or safely remove and isolate sharps and contaminated components. In developing such jigs, the designer inherently considers and embeds disassembly into the product. Secondly, some basic rules should be followed:
i. Avoid adhesives between components of different materials, unless creating a reusable module (and, even then, ideally not).
ii. Avoid overmoulds, if possible – fine for a few cycles of reuse, but ultimately these prevent recycling at end of life due to the mix of inseparable materials.
iii. Use a minimal variety of materials to reduce recycling complexity.
iv. Device robustness and durability should be maximised, even if cost is increased as a result (offset by reduced production volumes), to extend product/component lifetimes.
v. Furthermore, research is ongoing into technologies generally termed “active disassembly”. This proposes the use of elastomeric materials in fasteners that collapse under increased atmospheric pressure9 or shape memory alloys/smart polymers to neutralise a fastener under increased temperatures10 – both enabling rapid disassembly of a product. As the demand for circularity increases, these technologies are likely to mature rapidly.
d. Design for Reassembly: the main consideration here is that components may be assembled several times over their lifetimes. This may mean ensuring that snaps do not come close to their elastic limit or that screws and screw bosses are carefully selected and designed for multiple insertions without stripping. A device reassembly line would probably need to be integrated into the original assembly plant for the transfer of components, fixtures and operator skills to ensure consistency of quality.
e. Design for Sterility: ensuring the sterility of a device (or certain aspects of a device) from manufacture to patient is critical for many medical products. Is it possible to maintain this with a remanufactured device? As identified above, it should be possible to sterilise components and modules that are being reintegrated into new devices, with clean room procedures followed just as for new device manufacture. But what about packaging?
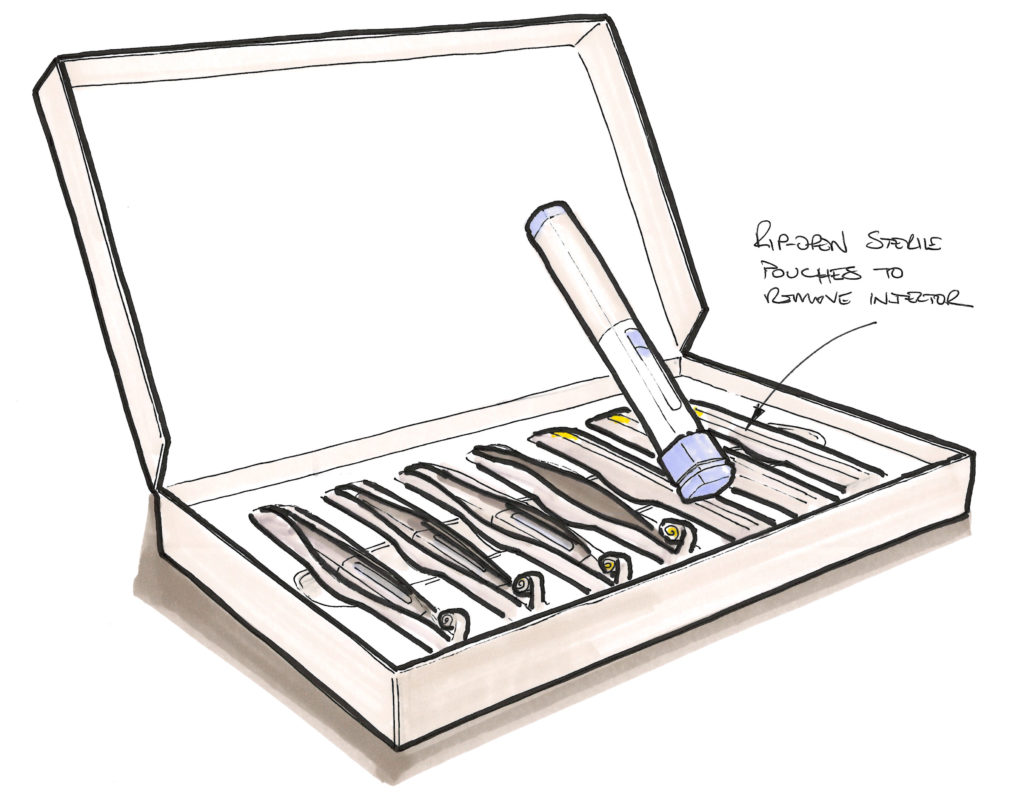
Figure 3: Sketch of sterile autoinjector packaging suggestion.
We are all familiar with sterile barrier pouches used to protect items such as lines, syringes, catheters and so on. These can now be made from highly recyclable single materials, such as PET or high-density polyethylene – for example, the 100% PET blister pack developed by RotorPrint (Olost, Spain) – and kept in a closed loop. But that relies on the end user returning the material to the system (alongside the device), when the more likely outcome will be that it is put in the waste and sent to landfill. One option to avoid this is to integrate the sterile barrier into the delivery/return box concept – this would add complexity to the design of the packaging but remove the separate material issue. Imagine a sterile pouch mechanically trapped within the card delivery box; the pouch could have a pull tag to rip it open along a weakness line, similar to an Amazon delivery envelope. Thus, the device is removed from its sterile barrier but the barrier itself is retained, returned, separated and recycled along with the box. Another option – not possible in all cases, admittedly, and complex in others – is to design out the packaging by integrating the sterile barrier into the product itself. For instance, a cap or cover on the product that maintains a hermetic seal around the sensitive components but is itself reusable upon return and sterilisation (Figure 3).
A WORD ON ELECTRONICS
By mass, the emissions footprint of a PCB assembly and associated components is 10–20 times that of a plastic component;11 therefore the integration of electronics into traditionally “dumb” or purely mechanical devices has immediate implications for that product’s footprint. However, this should not necessarily be looked at in isolation, especially as the development of connected health picks up speed. If the inclusion of electronics into a medical device prompts the patient to medicate correctly, or otherwise aids disease control and treatment development through data provision to the healthcare provider, this can lead to the avoidance of far greater emissions downstream associated with hospital admission or other interventions.
Phillips-Medisize provided an excellent example analysis of this in ONdrugDelivery, Issue 139 (Nov 2022),12 looking at connected asthma inhalers. For the treatment of asthma, two inhalers are typically prescribed – a preventer and a reliever. The former is to be taken regularly and aims to keep the disease under control. The latter is to be taken upon the onset of symptoms. However, it is quite common for people to disregard the preventer and rely solely on the reliever; thus the disease is not managed correctly, resulting in unnecessary hospital admissions. The resultant emissions associated with patient travel, hospital power, waste, water, etc outweigh those of a small PCB integrated into a preventer inhaler that would prompt the patient (directly, or via connectivity with their HCP) to manage their condition correctly.
Such a PCB could be recovered, reset and reintegrated into a new device in a circular process, further reducing its footprint. It might perhaps have a lithium-ion battery that needs recharging, or it could use one of the more sustainable battery solutions coming on stream if a non-rechargeable cell is required. Fuelium (Barcelona, Spain) is one such company pioneering sustainable battery solutions.
REFRAMING THE PROBLEM
Rather than trying to make existing designs and approaches fit the circular economy, there might be opportunities to reduce emissions in other ways. Sticking with inhalers: the propellant gas used in metered dose inhalers (MDIs) – those typically used by asthma patients – is a hydrofluorocarbon, which typically has a global warming potential tens or even thousands of times that of CO2.13 As such, MDIs contribute a massive 3% to the entire emissions footprint of the NHS.3 Because the propellant is expelled into the atmosphere with the medication, this cannot be returned and reused within a circular model.
The obvious approach, therefore, is to transition to low-carbon inhalers, of which there are several types already available, generally in dry powder form, but they only occupy a very small portion of the market. So, if an inhaler manufacturer wanted to pursue a wet inhaler but without the propellant emissions, it would have two choices:
a. Develop an emissions-free propellant gas to maintain existing technology
b. Design out the propellant.
The first route involves chemistry, experimentation and long clinical trials to ensure that the new propellant is not harmful when inhaled. The second is a design challenge that could yield further benefits, such as faster time to market and integration into the circular economy.
How do you design the propellant out of a system that relies on a propellant? The key here is the circular economy itself. Current inhalers are comprised of two simple plastic mouldings and a sealed, pressurised cartridge incorporating a metering valve – mass producible for minimal cost on an enormous scale but destined for landfill. By moving to a circular product-as-a-service system, complexity and cost of the device can increase as production volumes decrease. This paves the way for, by way of a hypothetical example, a pumped-air system: in such a device, the user might apply a few depressions to a large button (in lieu of the top of the cartridge) to pressurise a chamber. A visual indicator displays when sufficient pressure has been reached, and a one-way valve prevents over-pressurisation. A trigger then releases the air from the chamber, into which a metered dose of medication is injected (driven by the same air pressurisation, the mechanical action of button depression or the Venturi effect) through an aerosolising nozzle in the mouthpiece to the patient as per the current devices. The pressure indicator could act as an interlock for the trigger, preventing low-pressure activation. Complex? Yes, compared with current inhalers. Unforeseen issues? Probably – that is the nature of research and development. Impossible? No – there is no groundbreaking new science or technology here. Component count might be high and accuracy important but, with many of these components directly reusable, this should not be a barrier.
CONCLUSIONS
The challenges to making medical and pharmaceutical products truly sustainable are not insignificant – but not insurmountable either. Transitioning to the circular economy is essential, and there are some significant business and logistical steps to be taken by the industry – and manufacturers in particular – to establish successful systems of reclaim and remanufacture. Collaboration between all facets of the industry, including new providers not traditionally involved, will be necessary. But many of the inherent challenges are readily solvable through good design, by influencing user behaviour, designing for disassembly and reuse or entirely changing the established nature of a device. Once a product-as-a-service model has been implemented and embedded as a standard business model within a company, then solutions will always be found as an imperative to make the system as efficient and cost effective as possible – that is just the nature of business. The future is circular – and good design will get us there.
REFERENCES
- “Designing A Net Zero Roadmap For Healthcare”. Arup, Jul 2022.
- “Delivering a Net Zero National Health Service”. NHS England, Jul 2022.
- “Extended Producer Responsibility”. OECD web page, accessed Apr 2023.
- “Plastic Recycling”. British Plastics Federation web page, accessed Apr 2023.
- “Circular economy roadmap”. Philips web page, accessed Apr 2023.
- “ReturPen”. Novo Nordisk web page, accessed Apr 2023.
- Clews G, “Inhaler recycling scheme that cut carbon emissions equivalent to more than 8,500 cars is scrapped”. Pharm J, Jul 3, 2020.
- “What is Machine Vision?”. Cognex web page, accessed Apr 2023.
- Peeters JR et al, “Pressure-sensitive fasteners for active disassembly”. Int J Adv Manuf Tech, 2016, Vol 87, pp 1519–1529.
- “Active Disassembly using Smart Materials”. Web page, accessed Apr 2023.
- “Greenhouse gas reporting: conversion factors 2022”. Gov.uk web page, accessed Apr 2023.
- Simpson I, Bhandari V, “Considering The Sustainability Impact Of Connected Inhalers In The Treatment Of Asthma”. ONdrugDelivery, Issue 139 (Nov 2022), pp 42–46.
- “Guidance for Industry: Fluorinated gases (F gases)”. Gov.uk, Feb 2019.