Citation: Lumay G, Tripathi NM, Francqui F, “How to Gain a Full Understanding of Powder Flow Properties, and the Benefits of Doing so”. ONdrugDelivery Magazine, Issue 102 (Nov 2019), pp 42-46.
Geoffroy Lumay, Naveen Mani Tripathi and Filip Francqui show how the association of three recently developed flow measurement techniques – improved angle of repose measurement with GranuHeap; cohesiveness measurement with GranuDrum; and improved tapped density measurement with GranuPack – can be used to gain valuable insights into powder characteristics and properties.
INTRODUCTION
Granular materials, fine powders and nanostructured powders have wide applications in pharma1-4 including in oral tablets and capsules, and in inhalation. A robust manufacturing process involving powder requires reliable powder-flow properties. Unfortunately, pharmaceutical powders are usually cohesive and so a deep understanding of the forces acting between the grains is necessary.
Moreover, post-processing methods are commonly used to reduce cohesiveness.5,6 Powder behaviour is influenced by:
- steric repulsions
- friction forces
- cohesive forces, and
- interaction with the surrounding gas.7,8
“Spray-dried powders can be characterised before and after granulation with recently developed measurement devices to predict the processability.”
Steric repulsion is related to the grain geometry. Friction forces are influenced by both surface state (rough or smooth surface) and the chemical nature of the grains.
Cohesive forces may be induced by the presence of liquid bridges,9,10 by electrostatic charges,11-14 by van der Waals interactions15 or, more rarely, by magnetic dipole-dipole interactions.16 The predominance of one of these forces depends on both environmental conditions and the physicochemical properties of the grains.
Here, we show how spray-dried powders can be characterised before and after granulation with recently developed measurement devices to predict the processability. According to the spray-drying method, in particular according to the nozzle characteristics, the obtained powder is processable or not. We discuss how the granulation step modifies powder flow behaviour. In particular, we focus on two granulation methods: high shear granulation (HSG) and fluid-bed top-spray granulation (FBG).
EXPERIMENTAL METHOD
Spray-Drying
Amorphous solid dispersion (ASD) was obtained by spray-drying a 10% (w/w) ethanol solution containing 20% indomethacin (as model drug) and 80% polyvinylpyrrolidone (PVP)-K30. Spray-drying was performed using a ProCepT (Zelzate, Belgium) spray-dryer, using two different nozzles, bi-fluid and ultrasonic, in order to obtain different particle sizes.
Granulation
Wet granulation was performed by FBG and HSG (both ProCept machines). In order to obtain a good granulation process, 50% microcrystalline cellulose was added to the spray-dried powder. As granulation liquid 5% (w/w) PVP-K30 in ethanol was used. Fluid bed the temperature was kept low (product temperature 30°C) to avoid an additional negative effect on the ASD. HSG was more efficient compared with FBG as only 0.5% of PVP-K30 was needed to obtain a strong granule whereas with FBG 3.8% PVP-K30 was needed to obtain a granule with similar characteristics. In order to obtain a product with a similar ethanol content, the granules obtained by FBG were additionally dried for 1 hour in the fluid bed.
As the amount of granulation liquid needed is lower during HSG, it should be the preferred wet granulation technology to granulate ASDs. However, in all cases the ASD was maintained with a Tg of 110°C. So the addition of the ethanol (and increased temperature) had no influence on the ASD.
“The repose angle refers to the angle of the isosceles triangle with the same projected surface as the powder heap. The lower the repose angle is, the better the powder flowability.”
Angle of Repose (GranuHeap)
The GranuHeap instrument carries out an automated repose angle measurement technique based on image processing.6 A powder heap is created on a cylindrical support to be analysed by image processing. In order to obtain reproducible results, an initialisation tube with an internal diameter equal to the circular support is installed on the support. After filling the initialisation tube by hand with a fixed volume of powder (100 mL in the case of the present study), the initialisation tube moves up at a constant speed of 5 mm/s. Thereby, the powder is flowing from the tube to form a heap on the cylindrical support, which is then evaluated by image analysis.
In the present study, 16 images separated by a rotation of 11.25° were recorded. A custom image recognition algorithm determines the position of the powder/air interface. The repose angle refers to the angle of the isosceles triangle with the same projected surface as the powder heap. The lower the repose angle is, the better the powder flowability.
Dynamic Cohesive Index (GranuDrum)
GranuDrum is an automated powder flowability measurement instrument that uses the rotating drum principle.6,7 A horizontal cylinder with vertical glass sidewalls, the drum, is half filled with sample powder. For the present study, the drum rotates around its axis at an angular velocity from 2-10 rpm. A charge coupled device camera takes snapshots (50 images separated by 0.5 s) at each angular velocity. The air/powder interface is detected on each snapshot with an edge detection algorithm. Afterwards, the average interface position and the fluctuations around this average position are computed. Then, for each rotation rate, the flow angle is computed from the average interface position and the dynamic cohesive index is measured from the interface fluctuations.
Interface fluctuations are induced by the cohesive forces between the grains. The dynamic cohesive index is close to zero for non-cohesive powders and increases when the cohesive forces intensify. In addition, this method gives the opportunity to study complex rheological properties of powders (shear thinning, shear thickening and thixotropic behaviour) by varying the rotation rate.
Tapped Density (GranuPack)
GranuPack conducts automated and improved tapped density measurement.6 The Hausner ratio Hr (or the Carr index), the initial density ρ0 and the final density ρ500 are measured precisely. Moreover, dynamic information and an extrapolation of the optimum density (ρ∞) can be extracted from compaction curves. The compaction curve is a plot of the bulk density as a function of the tap number. At the beginning of the measurement, the powder is placed in a metallic tube with a rigorous initialisation process. Afterwards, a light hollow cylinder is placed on the top of the pile to keep it flat during compaction. A single tap consists of moving up the tube containing the powder sample to a height (ΔZ) of 1 mm and then performing a free fall. The free-fall height ΔZ can be adjusted. The height h of the powder bed is measured automatically after each tap.
From the height (h), the volume (V) of the bed is computed. As the powder mass (m) is known, the bulk density ρ is evaluated automatically and plotted after each tap. The measurements are performed with 35 mL of powder subjected to 500 taps.
Size Distribution
The particle size distribution of the spray-dried powders was obtained by dry powder laser diffraction. Powders were dispersed with compressed air at 1.5 bar through a RODOS dry disperser before sizing with a HELOS laser diffraction sensor (measurement range R1: 0.18 – 35 μm and R3: 0.5-175 μm) (all from Sympatec, Etten-Leur, The Netherlands). The particle size distribution of the granules was evaluated by dynamic image analysis with QicPic granulo-morphometer (Sympatec). The granules were gravimetric dosed via the GRADIS disperser.
MATERIALS
Four powders with different granulometries were created by spray-drying. The powders and the associated production process are listed in Table 1. For all powders, a complete amorphous system was created. Powder P1 was spray-dried with a small cyclone, 0.2 mm nozzle and an air flow-rate of 5.5 L/min at 2.75 bar. Powder P2 was spray-dried with a large cyclone, 0.6 mm nozzle and an air flow rate of 8 L/min at 0.41 bar. Powder P3 was spray-dried with large cyclone, 0.6 mm nozzle and an air flow rate of 4.1 L/min at 0.41 bar. Finally, powder P4 was spray-dried with an ultrasonic nozzle at a frequency of 25 kHz at 35%.
Name | Short Name | Process | Information |
Powder1 | P1 | Spray-drying | Bi-Fluid nozzle |
Powder2 | P2 | Spray-drying | Bi-Fluid nozzle |
Powder3 | P3 | Spray-drying | Bi-Fluid nozzle |
Powder4 | P4 | Spray-drying | Ultrasonic nozzle (25KHz) |
Granules1 | G1 | High shear granulation (HSG) | with Powder2 |
Granules2 | G2 | High shear granulation (HSG) | with Powder4 |
Granules3 | G3 | Fluid bed top spray granulation (FBG) | with Powder4 |
Table 1: Powders and granules used in the study and corresponding production processes.
The granules were created starting from powders P2 and P4. The reasons of this selection will be discussed hereafter. G1 granules were produced with HSG starting from powder P2. G2 and G3 granules were produced starting with powder P4 using HSG and FBG, respectively.
RESULTS & DISCUSSION
Particle Sizes
The main parameters extracted from grain size distributions are summarised in Table 2. Both powders and granules cover a wide range of grain sizes. Compared with the spray-dried powder, the particle size increased for all granules combined with a decrease of the span, indicating a narrower particle size distribution. Powder P4, which is directly processable, has the highest grain size among the spray-dried powders. The granules obtained by HSG (G2) or FBG (G3) of powder P4 resulted in a similar particle size distribution.
Short Name | D10 (μ) | D50 (μ) | D90 (μ) | Span |
P1 | 0.63 | 3.33 | 8.11 | 2.25 |
P2 | 1.87 | 9.25 | 24.73 | 2.47 |
P3 | 3.81 | 18.48 | 46.08 | 2.29 |
P4 | 16.09 | 48.05 | 116.20 | 2.08 |
G1 | 41.73 | 97.99 | 209.32 | 1.71 |
G2 | 87.12 | 252.79 | 475.29 | 1.54 |
G3 | 154.63 | 291.39 | 516.46 | 1.24 |
Table 2: Summary of grain size distribution parameters of powders and granules studied.
Repose Angle
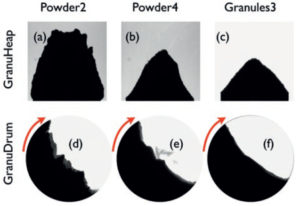
Figure 1: Typical pictures of heaps and of the flow inside the rotating drum with two powders (P2 and P4) and with G3 granules.
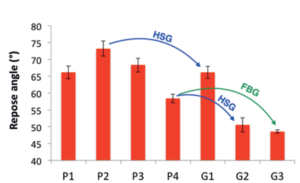
Figure 2: Repose angle measured with GranuHeap. The measurement is repeated three times to perform an average and the error bars correspond to the standard deviation.
Figure 1 shows typical pictures obtained with GranuHeap. One can see that the different powders have qualitatively very different behaviours and that the flowability of granules is better. The quantitative results obtained with GranuHeap are shown in Figure 2. Globally, the repose angle decreases when the grain diameter increases. The powder P1 is found to be an exception to this general rule because this fine powder has the tendency to form agglomerates. The repose angle decreases significantly after granulation, showing the better flowability of granules. Among the spray-dried powders, powder P4 has the lower repose angle.
Error bars corresponding to the standard deviation over three repose angle measurements are relatively small compared with the differences between the samples. This good reproducibility is obtained thanks to GranuHeap being automated and its use of a camera.
Based on the repose angle results obtained with the powders, the choice was made to granulate two of them having respectively bad and good flow behaviour.(This was the reason we choose to granulate powder P2 and powder P4 by both HSG and FBG.) However P2 seemed to be too fine to be granulated in the fluid bed.
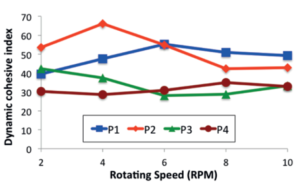
Figure 3: Dynamic cohesive index of the powders measured from the fluctuations of the flow in GranuDrum.
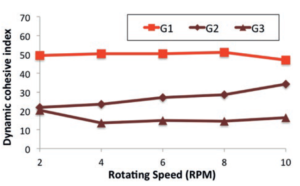
Figure 4: Dynamic cohesive index of the granules measured from the fluctuations of the flow in GranuDrum.
Dynamic Cohesive Index
The angle of repose gives a static and straightforward picture of a powder’s flow properties. If rheological information is needed, flow measurements at different shear rates or different speeds are needed.
The cohesiveness is measured with GranuDrum at different speeds for the powders (see Figure 3) and for the granules (see Figure 4).
Globally, Powder P2 has the higher cohesiveness and powder P4 has the lowest cohesiveness. However, the cohesiveness of powder P2 decreases with the rotating speed, showing a shear thinning behaviour generally due to aeration. This kind of behaviour could be interesting for some processes and could also cause complications (dosage fluctuation for example) due to unexpected variations of the flowing properties. Typically, a low and constant cohesiveness is recommended.
The G1 granules obtained from granulation of powder P2 show a constant cohesiveness slightly lower than the cohesiveness of the powder. The decrease of cohesiveness is more important for G2 and G3 granules obtained by granulation of powder P4. The dynamic cohesive index measurements also show that powder P4 has the lowest cohesiveness compared with other spray-dried powders.
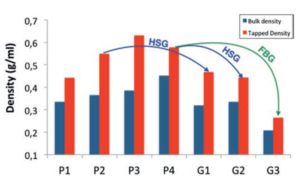
Figure 5: Bulk and tapped densities obtained with the automatic measurement
instrument GranuPack.
The bulk and tapped densities of the granules are lower than those of the powders (see Figure 5). Typically, the density decreases with the cohesiveness. In the present case, the decrease of the density after granulation is attributed to the modification of the size distribution width (Span).
It is well known that a granular material having a larger span (as the spray-dried powder) has a higher density because the small grains fill the gaps between the big grains. The GranuPack results arise due to competition between cohesive forces and geometric (grain size/shape) effect.
CONCLUSION
Depending on the spray-drying process (nozzles and airflow rates), different grain sizes are produced leading to different flowing and packing behaviours. The powder P4 obtained with the ultrasonic nozzle shows good flow indexes (low angle of repose, low cohesiveness and relatively high density). The other powders have a lower average grain sizes and consequently worst flow properties.
A selection of two spray-dried powders (best and worst flowing properties) was granulated with both HSG and FBG. Globally, granulation improves the powder flow properties. The effect is particularly well evidenced by the angle of repose measured with the automated GranuHeap instrument.
Moreover, the rotating drum measurements (GranuDrum) shows that the flow properties of the granules are more stable while the powders have more complex rheological behaviour. Finally, the GranuPack instrument shows significant differences of bulk and tapped densities before and after granulation. These differences are the result of interplay between the effect of the cohesive forces and of the grain size distribution width.
ACKNOWLEDGEMENT
At the University of Liège, this study is conducted in the framework of the “PowderReg” project, funded by the European programme Interreg VA GR within the priority axis 4 to: “strengthen the competitiveness and the attractiveness of the Grande Région / Großregion”.
REFERENCES
- Muzzio F, Shinbrot T, Glasser B, “Powder technology in the pharmaceutical industry: the need to catch up fast”. Powder Tech, 2002, Vol 124, pp 1-7.
- Wu C-Y, Dihoru L, Cocks A, “The flow of powder into simple and stepped dies”. Powder Tech, 2003, Vol 134, pp 24-39.
- Ruenraroengsak P, Cook J, Florence AT, “Nanosystem drug targeting: Facing up to complex realities”. J Controlled Release, 2020, Vol 141, 265.
- Van Snick B et al, “A multivariate raw material property database tofacilitate drug product development and enable in-silico design of pharmaceutical dry powder processes”. Int J Pharmaceutics, 2019, Vol 549, pp 415-435.
- Shanmugam S, “Granulation techniques and technologies: recent progresses”. Bioimpacts, 2015, Vol 5, pp 55-63.
- Mahdi F, Hassanpour A, Muller F, “An investigation on the evolution of granule formation by in-process sampling of a high shear granulator”. Chem Eng Res Design, 2018, Vol 129, pp 403-411.
- de Gennes P, “Granular matter: a tentative view”. Rev Mod Phys,1999, Vol 71, S374.
- Lumay G et al, “Measuring the flowing properties of powders and grains”. Powder Tech, Vol 224, pp 19-27.
- Boschini F et al, “Linking flowability and granulometry of lactose powders”. Int J Pharmaceutics, 2015, Vol 494, pp 312-320.
- Lumay G et al, “Effect of relative air humidity on the flowability of lactose powders”. J Drug Del Sci Technol, 2016, Vol 35, pp 207-212.
- Schella A, Herminghaus S, Schroter M, “Influence of humidity on tribo-electric charging and segregation in shaken granular media”. Soft Matter, 2017, Vol 13, pp 394-401.
- Rescaglio A et al, “Combined effect of moisture and electrostatic charges on powder flow”. EPJ Web of Conferences, 2017, Vol 140, 13009.
- Matsusaka S et al, Triboelectric charging of powders: A review”. Chem Eng Sci, 2010, Vol 65, pp 5781-5807.
- Šupuk E et al, “Tribo-electrification of active pharmaceutical ingredients and excipients”. Powder Tech, 2012, Vol 217, pp 427-434.
- Capece M et al, “On the relationship of inter-particle cohesiveness and bulk powder behavior: Flowability of pharmaceutical powders. International”. J Pharmaceutics, 2016, Vol 511, pp 178-189.
- Lumay G et al, “Flow of magnetized grains in a rotating drum”. Phys Rev, 2010, E82, 040301.