To Issue 135
Citation: Neveu A, Patel D, Francqui F, “Influence of Additives on the Electrostatic Charge Build-Up of Excipients”. ONdrugDelivery, Issue 135 (Jul 2022), pp 12–15.
Aurélien Neveu, Devang Patel and Filip Francqui present a study investigating the influence of additives on the tribocharging behaviour of two excipients using Granutools’ GranuCharge instrument.
Good flowability is a key factor for numerous powder processes, with the consistency of the flow directly related to the final product quality. However, when a powder flows, the frictional contact between the particles creates electrostatic charges via the triboelectric effect. This charge build up induces an increase in the strength of the electrostatic cohesive interactions that contribute to the global cohesiveness of the powder and therefore a decrease in its flowability properties.
Moreover, charged particles tend to stick to the surface of pipes and machine parts. A non-negligible amount of material can thus stay trapped in the conveying device, clog pipes and induce undesirable variability of the mass flow. In a 2021 study, Allenspach et al1 demonstrated the correlation between the propensity of powders to accumulate charge during their flow and the sticking of particles at the output of a loss-in-weight feeder. They demonstrated that high charge density leads to heavy sticking and irregular flow, and thus a significant overshoot of the target mass flow. Therefore, the relationship between the sensibility of a powder to tribocharging is directly related to its processing performance.
“Reducing the amount of additives is usually beneficial for reducing production costs and easier regulatory compliance.”
The addition of additives is a common procedure in the pharmaceutical industry to improve the properties of an excipient and API blend. For example, mesoporous silica has been shown to significantly improve the flowability of excipients by both reducing the capillary bridges between the particles and producing a conductive network to efficiently dissipate the charges throughout the material.2 However, additives of different nature are generally used and a systematic evaluation of their influence on the electrostatic behaviour of the blend is still lacking.
In this study, the influence of three common additives – Syloid® S244, Magnesium Stearate (MgSt) and Aerosil® 200 – on the tribocharging properties of two excipients– microcrystalline cellulose (MCC), Starch 1500® – was investigated. The tribocharging characteristics of the blends were evaluated with the GranuCharge instrument (Granutools), which evaluates the charge build-up due to the flow through a set of SS316L pipes.3
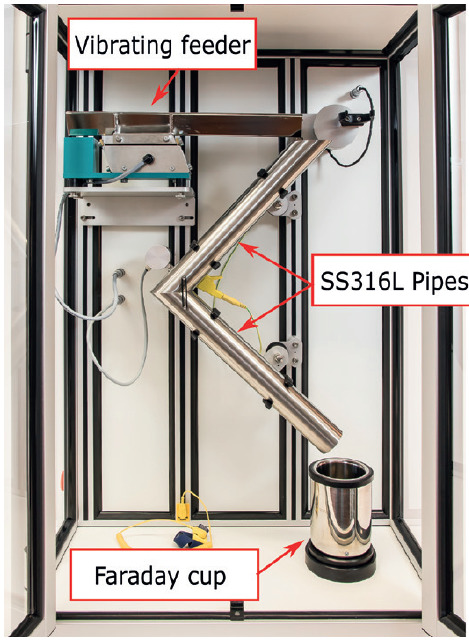
Figure 1: GranuCharge instrument (Granutools).
EXPERIMENTAL METHOD
The tribocharging properties of the selected excipients were investigated with GranuCharge (Figure 1). The main component of this device is the Faraday cup, specifically designed to measure low electrostatic charges in powders or granular materials. A set of V-shape pipes is used to evaluate the tribocharging sensibility of the powder during a flow. Numerous pipe materials are available (PVC, teflon, copper, etc.) but stainless steel (SS316L) pipes were used in this study. Both the pipes and the operator were grounded during the measurement to avoid influencing the powder charge. A vibrating feeder was used to pour the powder into the pipes.
The measurement protocol was as follows. First, the initial charge density was determined, as it depends on the history of the powder (production, transport, handling), and used as a reference to evaluate the charge build-up after the flow through the pipes. After measuring out 55 mL of the powder, the initial charge density (q0) was measured by pouring the powder directly into the Faraday cup. Then, the powder was extracted from the cup and placed in the vibrating feeder. The sample then flowed through the V-shape stainless steel pipes into the Faraday cup, allowing measurement of the final charge density (qf). The charge density variation (Δq = q0-qf) due to the gain or loss of electrostatic charges during the flow was then determined. The measurement was repeated three times on fresh (unused) samples to assess repeatability. In this study, the average computed over the three tests was reported, with the error bars corresponding to the standard deviation around the mean.
MATERIALS
Two excipients (MCC, Starch 1500®) and three additives (Syloid® S244, MgSt, Aerosil® 200) were selected for this study. The blends were produced for each excipient/additive combination for 0.5, 1 and 2% w/w of additives. Therefore, the effect of each additive/exception combination can be evaluated for the tribocharging properties, as well as the influence of the amount of additive. Each excipient additive combination blend was prepared by passing excipient through a #25 mesh screen and additive through a #60 mesh screen and then blended in a V-Blender at 15 RPM for 5 minutes. The resultant blend was then used for further study.
RESULTS AND DISCUSSION
The obtained initial and final charge densities, as well as the charge build-up, are reported in Table 1 for all excipient/additive combinations. The charge density measured for the raw excipient (without additives) is also shown. The final charge density, obtained after the flow through the stainless steel pipes, is presented in Figure 2. After the flow, the two raw excipients show a high charge density of about -5 nC/g for the MCC and -4 nC/g for the starch. The two excipients demonstrate a high sensibility to tribocharging, which is likely to induce electrostatic-related processability problems. Every combination of excipient/additive leads to a reduction of the final charge density. This reduction can be associated either with a decrease in electrostatic charge generation or a drastic increase in the global conductivity of the material, allowing a more efficient charge dissipation.
Name | q0 (nC/g) | qf (nC/g) | Δq (nC/g) | Name | q0 (nC/g) | qf (nC/g) | Δq (nC/g) |
STARCH1500 | -6.62 x 10-1 | -3.49 | -2.83 | MCC | -4.17 x 10-1 | -4.41 | -4.00 |
Starch + S244 0.5% | -5.61 x 10-1 | -9.70 x 10-1 | -4.08 x 10-1 | MCC + S244 0.5% | -5.99 x 10-1 | -2.45 | -1.85 |
Starch + S244 1% | -5.91 x 10-1 | -5.54 x 10-1 | 3.66 x10-2 | MCC + S244 1% | -1.02 | -1.71 | -6.86 x 10-1 |
Starch + S244 2% | -6.14 x 10-1 | -3.36 x 10-1 | 2.77 x 10-1 | MCC + S244 2% | -8.12 x 10-1 | -8.69 x 10-1 | -5.72×10-2 |
Starch + Mg St 0.5% | -6.03 x 10-1 | -1.73 | -1.12 | MCC + Mg St 0.5% | -7.21 x 10-1 | -2.79 | -2.07 |
Starch + Mg St 1% | -4.37 x 10-1 | -1.18 | -7.46 x 10-1 | MCC + Mg St 1% | -9.41 x 10-1 | -2.25 | -1.31 |
Starch + Mg St 2% | -2.67 x 10-1 | -3.54 x 10-1 | -8.72×10-2 | MCC + Mg St 2% | -4.53 x 10-1 | -1.79 | -1.34 |
Starch + Aer 0.5% | -6.50 x 10-1 | -6.97 x 10-1 | -4.62×10-2 | MCC + Aer 0.5% | -6.40 x 10-1 | -9.24 x 10-1 | -2.84 x 10-1 |
Starch + Aer 1% | -4.80 x 10-1 | -6.99 x 10-1 | -2.19 x 10-1 | MCC + Aer 1% | -4.09 x 10-1 | -3.79 x 10-1 | 3.02×10-2 |
Starch + Aer 2% | -3.22 x 10-1 | -1.03 | -7.08 x 10-1 | MCC + Aer 2% | -3.59 x 10-1 | -9.67×10-2 | 2.63 x 10-1 |
Table 1: Initial charge density (q0), final charge density (qf) and total charge build-up (Δq) measured with the GranuCharge for the different blends.
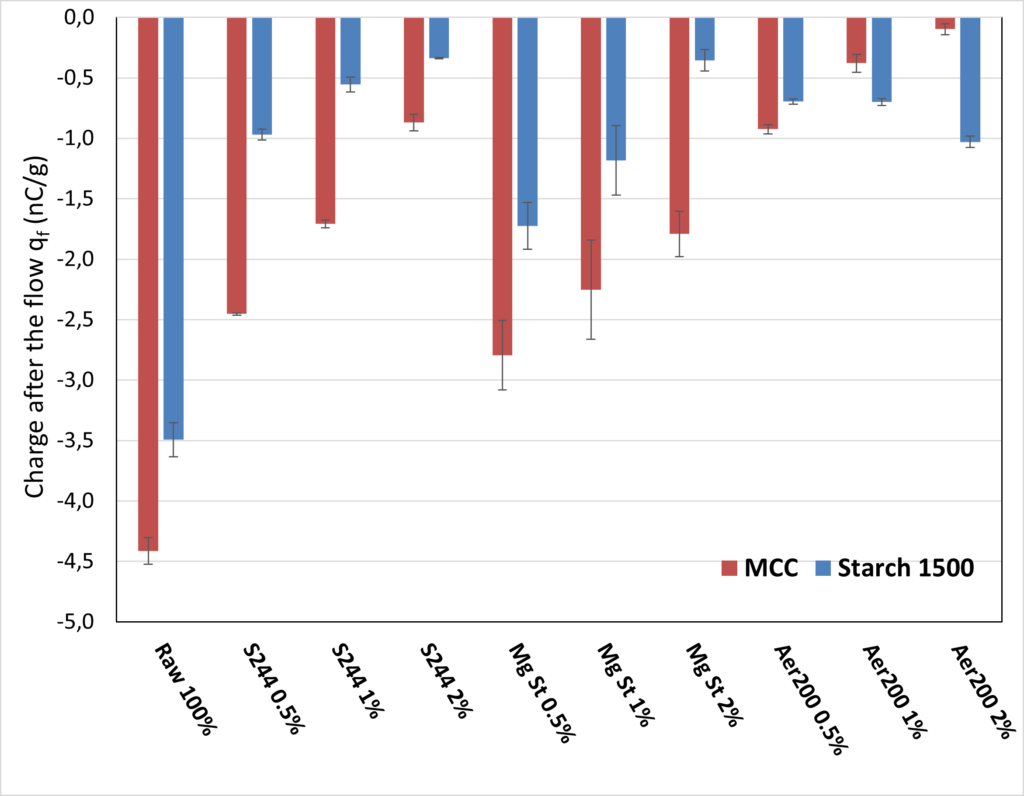
Figure 2: Charge density measured after the flow through the SS316L pipes for different %w/w of the three additives.
To highlight the beneficial influence of the additive, the charge build-up reduction (CBR) is presented in Figure 3. The CBR quantifies the amplitude of the reduction of the charge build-up due to the presence of the additive compared with the charge build-up obtained with only the raw material. Therefore, a CBR of 50% indicates that the charge build-up measured with the additive is 50% lower than the one measured for the excipient alone.
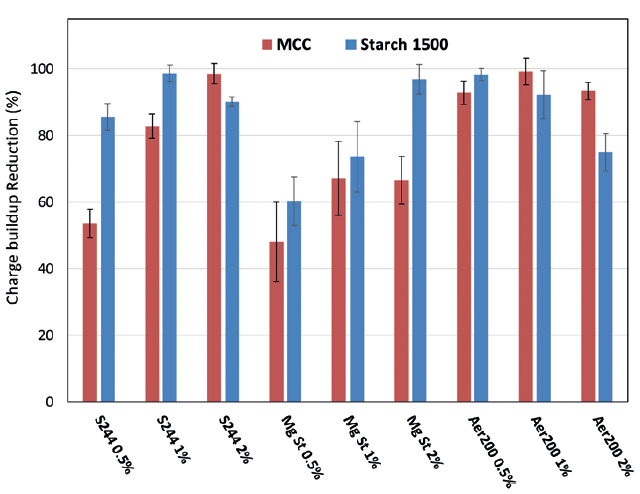
Figure 3: CBR for different %w/w of the three additives.
“These results highlight the importance of systematic characterisation of the influence of additives on the triboelectric behaviour of powder blends.”
A significant reduction of the charge build-up is observed with CBR >48% for all excipient/additive combinations. This confirms the usefulness of these additives in reducing the electrostatic charging of the blends. However, it was observed that the effect of the additives is not the same for the MCC and the Starch 1500. Indeed, the Syloid S244 shows a stronger CBR when associated with Starch 1500, resulting in at least CBR >80% for the three tested additives. An optimum CBR was obtained for 1% of Syloid S244, but then the effect decreased with increased content. Different behaviour was observed with the MCC, and a clear influence of the content of Syloid S244 was observed. At 0.5% of Syloid S244, the CBR was about 55% but drastically increases to 99% for 2% of Syloid S244.
The MgSt shows a lower CBR for both excipients, except for the Starch 1500 at 2% MgSt. For the MCC, a slight increase in CBR is observed from 0.5% to 1% MgSt but still a lower performance compared with the Syloid S244 and the Aerosil 200. For the Starch 1500, the CBR was strongly dependent on the additive content, with a constant increase up to 97% for 2% of MgSt. Therefore, the performance of the MgSt on the Starch 1500 at a content of 2% is similar to the Syloid S244 and the Aerosil 200.
Finally, the Aerosil 200 had a strong impact on the CBR. In particular, the MCC shows a CBR >90% for all additive content. Reducing the amount of additives is usually beneficial for reducing production costs and easier regulatory compliance. From these results, it can be determined that the Aerosil 200 is the additive that shows the higher CBR for MCC at the lower content (0.5%). However, for Starch 1500, the effect of the Aerosil 200 is different – at 0.5% of Aerosil 200, a high CBR is observed (>98%), but a subsequent increase of additive content leads to a decrease in the CBR.
CONCLUSION
In this study, the influence of three common additives (Syloid S244, MgSt, Aerosil 200) on the tribocharging behaviour of two excipients (MCC, Starch 1500) was investigated with GranuCharge. A significant reduction in the charge build-up of the blends was observed for all excipient/additive combinations. However, the additives can exhibit different performance in terms of charge reduction depending on the excipient with which they are associated. Furthermore, increasing the additive content does not systematically improve the charge reduction. In some cases, an optimum is observed and higher additive content leads to lower charge reduction.
These results highlight the importance of systematic characterisation of the influence of additives on the triboelectric behaviour of powder blends. Indeed, the complexity of the mechanisms involved in tribocharging powders makes it very difficult to predict what the charge build-up will be. Fortunately, the GranuCharge instrument has demonstrated its ability to evaluate the tribocharging ability of the different blends. This study is a preliminary work that opens up promising outcomes for future investigation. In particular, it is an ongoing study aiming to understand more deeply the interaction between the excipient and the additive particles that leads to the CBR.
REFERENCES
- Allenspach C et al, “Loss-in-weight feeding, powder flow and electrostatic evaluation for direct compression hydroxypropyl methylcellulose (HPMC) to support continuous manufacturing”. Int J Pharm, 2021, Vol 596, p 120259.
- Lumay G et al, “Influence of mesoporous silica on powder flow and electrostatic properties on short and long term”. J Drug Deliv Sci Technol, 2019, Vol 53, p 101192.
- Rescaglio A et al, “Tribo-electrification of pharmaceutical powder blends”. Part Sci Technol, 2019, Vol 37(8), pp 1024–1031.