Citation: Scherer M, “Expert View: Sharing is Caring: Polymer Suppliers as Medical Device Development Partners. ONdrugDelivery Magazine, Issue 100 (Sep 2019), pp 34-37.
Manuel Scherer looks at whether the advent of wearable injectors calls for a more collaborative approach between polymer suppliers and medical device developers.
“For many decades, the use of polymer resins in the medical device industry has brought significant value for the medical sector and enabled life-preserving treatments to the patients it serves.”
The advent of biologic drugs and wearable injectors for drug delivery presents new opportunities for plastic materials in the medical devices sector. A look at a few of the most significant trends influencing drug device development for high-viscosity injectable drugs provides insights into how the polymers industry is addressing such challenges. This article highlights the complex landscape of patient, converter and original equipment manufacturer (OEM) needs as well as considerations for polymer manufacturers in the development of new material formulations.
Designing a novel medical device to meet the high-end requirements of the evolving pharmaceutical drug delivery market requires the involvement of material specialists at an early stage to help identify materials that can maximise device performance and manufacturability whilst also addressing the important element of patient safety. Based on material engineering data, material specialists can identify potential device failure modes and provide design and material recommendations to support the development of high-performance medical devices.
POLYMERS: A STRONG TRADITION IN MEDICAL DEVICES
For many decades, the use of polymer resins (commonly referred to as plastics) in the medical device industry has brought significant value for the medical sector and enabled life-preserving treatments for the patients it serves.
Several major milestones indicate just how important developments in this area have been to modern patient care. Boehringer Mannheim revolutionised blood sugar measurement with its Reflolux® devices – the first series of blood glucose meter that enabled patients to measure their blood glucose level at home for the first time. In the early 1980s, Fresenius Medical Care launched the dialyser to treat kidney disease that featured plastic housing, end cap and polymer membrane. In the field of drug delivery, Novo Nordisk’s NovoLet® pen was amongst the first commercially available single-use injector pens to treat diabetes. What all of these examples have in common is that polymers enabled efficient large-scale production, mainly using injection moulding processing technology.
KNOWLEDGE INSPIRES UPTAKE AND INNOVATION
“Designing smaller devices is not only aligned with patient expectations but also represents another viable route to achieving carbon footprint reductions.”
Over time, knowledge of plastics, conversion technologies, secondary operations and predictive engineering capabilities has dramatically increased. This has allowed the device industry to enhance the user friendliness of their devices, integrating additional functionalities into subsequent device generations.1 Products have become smaller, easier to use, safer, and visually and haptically more appealing. The healthcare industry is risk averse, meaning that advances in healthcare devices have come largely using polymer materials that have been in use for many years in other industries with faster innovation cycles in place.
We are now entering a new era in care with a growing role for biopharmaceuticals, whereby modern-day techniques have made this class of protein-based drugs a more viabletreatment option. Biologics are medicines that are made or derived from a biological source and, as such, are complex macromolecules.
As they easily degrade in the digestive tract, biologics must be delivered by injection in one of three ways: subcutaneous, intravenous (IV) or intramuscular (IM). Their macromolecular nature causes their solutions to have a higher viscosity than those from small-molecule pharmaceuticals. When diluted down to practical viscosity levels, the required volume to be delivered calls for wearable devices that can do so in a controlled manner. Such devices typically contain more complex mechanical parts than, for example, an injector pen. This requires proper material selection, device design and an optimal industrialisation strategy in the early stages of new device development.
THE IMPLICATIONS OF THE SHIFT
What implications does the paradigm shift towards biologics have on device development and consequently on the plastic materials selected? Consider that biological drugs are large-volume molecules with significantly higher viscosities than small-molecule drugs.2,3 At the same time, the device industry is challenged to enable self-administration by the patient. This shifting in the point of care from the hospital environment to the home is driven in part by the need for authorities to reduce healthcare-related costs. These elements have consequences for device design, specifically in relation to push force and dosage accuracy.
COUNTERING AN ECOSYSTEM IMBALANCE
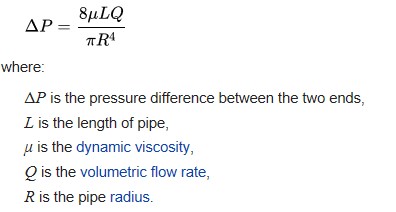
Figure 1: Hagen-Poiseuille equation.
As one can derive from the Hagen-Poiseuille equation (Figure 1), the main parameters influencing the push force that must be applied to perform the injection mechanism are viscosity, flow, needle diameter and length. One of the key targets in any new device development is to ensure that overall push force remains stable (or ideally decreases further) to make the injection as convenient as possible for the patient. The rise of biologics initially moves the ecosystem for push force out of balance.
Counteracting the increased viscosity of biological drugs with needle diameter or flow rate is not a recommended option because it results in increased pain and can trigger other negative side effects for the patient. Hence, other options should be considered during the design process to be able to cope with either the higher push force or the longer delivery time of a larger volume of liquid.
Integrating a mechanically loaded spring system into pen injectors has been a solution for more than a decade. Such a system reduces the activation or push force for a patient to unleash the injection mechanism. The higher viscosity of biologics has created a new challenge in this space, however, whereby significant plastic deformation can emerge from the higher spring loads. When coupled with prolonged storage times, this can lead to device failure.
OVERCOMING PLASTIC DEFORMATION
Typically, in autoinjectors and pen devices, spring loads are applied to the dosing mechanism to minimise the push forces for the patient. In the case of wearables where injections are performed over a longer time, mechanical gears and pump systems also come into play. This inherently brings static and dynamic loads on the materials used in the device over its lifetime, potentially resulting in plastic deformation, which may cause inaccurate dosage and/or fluctuating push forces.
Hence, materials with a proper impact/stiffness balance and, more importantly, creep and fatigue behaviour should be considered during the design phase to reduce the chances of failure. In this regard, different polymer families show different inherent properties. Specifically in relation to filled resins and irrespective of polymer type, anisotropic data is required for a predictable performance (Figure 2).4
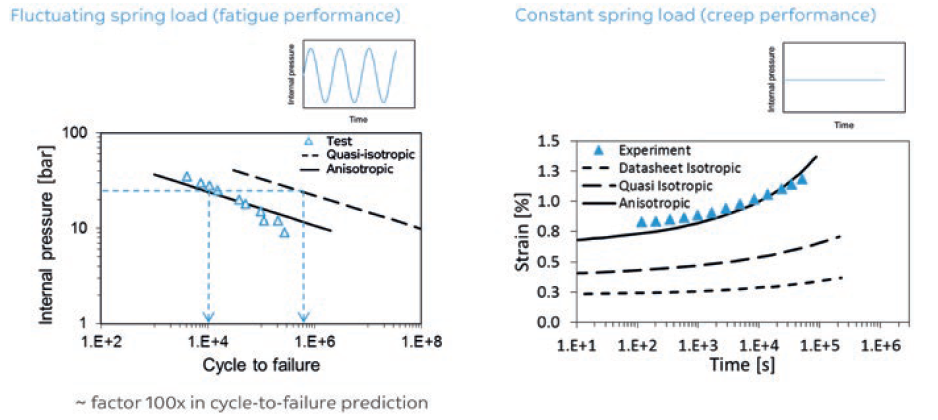
Figure 2: Creep and fatigue failure prediction models.
Besides increased mechanical loads, components involved in the drive mechanism will also be subject to movements. These movements should be smooth and accurate so as not to compromise dosage accuracy and push force. Internally lubricated high-performance materials with low co-efficients of friction, developed to overcome such specific tribological challenges, are being widely adopted by the device industry.
THE RISK OF OVERESTIMATION
Reinforced polymers are a viable option to design polymers for improved creep performance over the life of the device. However, an inherent consequence of designing devices with reinforced materials is anisotropy in the mechanical properties. Predictive engineering based on high-quality material data and computer-aided engineering (CAE) expertise is an essential step to mitigate failure modes and leverage device miniaturisation potentials.
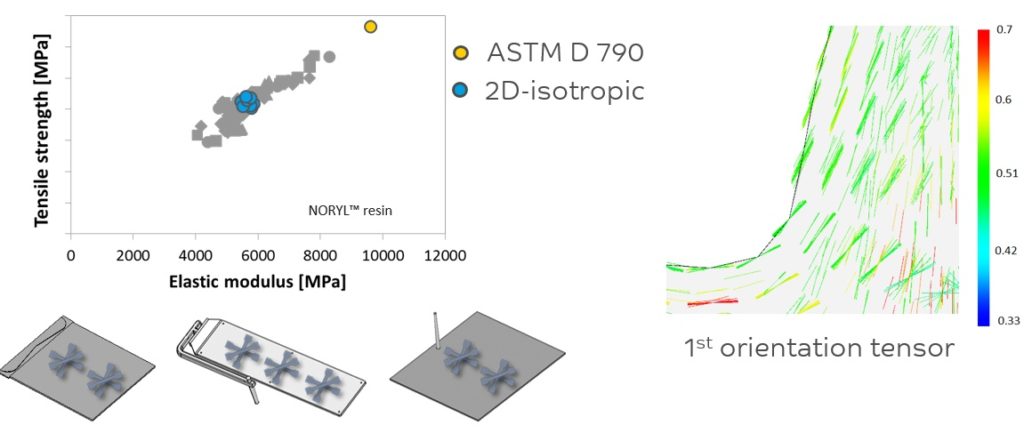
Figure 3: The effect of material anisotropy on the balance between tensile strength and elastic modulus.
Figure 3 illustrates the effect of material anisotropy on the balance between tensile strength and elastic modulus. Thorough process modelling methodology – combining Moldflow® (Autodesk, San Rafael, CA, US) Digimat® (Hexagon, Luxembourg) and Abaqus® (Abaqus, Johnston, RI, US) software – is employed to establish the processing-morphology-property relation in fibre-reinforced parts and predict their anisotropic mechanical performance.
The right-hand side of the figure illustrates how fibres may be oriented in an actual part based on predictive engineering techniques. The left-hand side shows the importance of using proper data for feeding the CAE tools for actual performance prediction. The grey dots describe the actual properties measured in three directions at two different locations in the sample plaques shown.
This data enables the best performance prediction. The blue dots average out those three measuring directions (0º, 45º, 90º) for the various samples and are an isotropic simplification of the real data. Using the datasheet values indicated by the yellow dot would lead to an overprediction of part performance. Only with the right expertise and accurate data can one come to the right predictions of performance and hence select the optimum material.
Figures 2 and 3 highlight the need for anisotropic data versus datasheet values. Anisotropic data is an important element in the design stage of a device, especially with regard to predicting long term static or dynamic failure modes. Relying purely on datasheet values in the design phase can increase the chance of designing too optimistically.
MINIATURISATION: SMALLER ALSO MEANS MORE SUSTAINABLE
Designing smaller devices is not only aligned with patient expectations5 but also represents another viable route to achieving carbon footprint reductions. However, practical considerations such as tool filling or stiffness – along with the integration of more functions (e.g. connectivity) and larger primary containers (a result of the paradigm shift of towards biologics) – represent natural limitations to the extent that downgauging can be realised.
In relation to tool filling, copolymer technologies exist which show significantly better rheological behaviour at high shear rates, despite the fact that they show identical flow per data-sheet values. This potential can be translated into wall-thickness reductions.
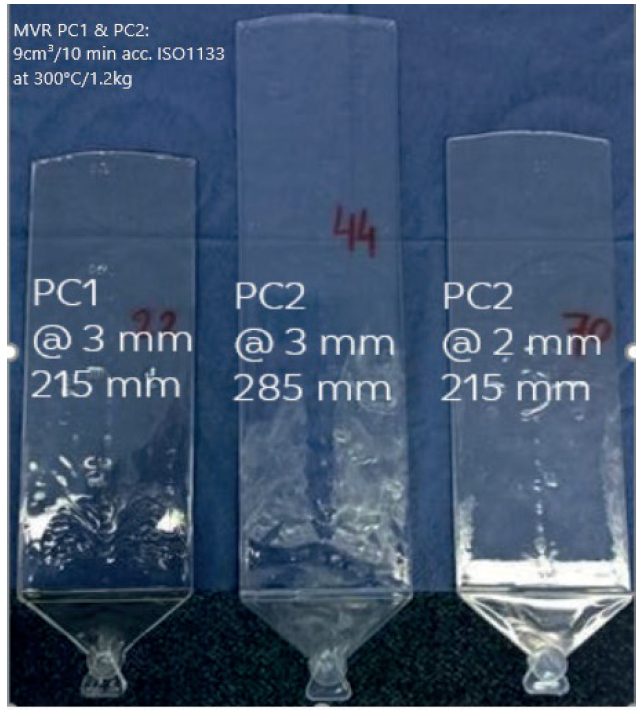
Figure 4: Materials with identical MVR on their datasheets processed at identical injection pressures show a different L/D flow behaviour.
Consider comparing two polycarbonates of identical flow, as per their datasheet values. As Figure 4 shows, in spite of the identical flow, polycarbonate copolymer PC2 achieves either a greater flow length orprovides a 1 mm wall thickness reduction over standard polycarbonate PC1, when processed under identical conditions. This is an implication of the molecular backbone of the material that enables increased flow length over wall thickness (L/D), specifically at the high shear rates that occur during injection moulding. This feature can be used to miniaturise or downgauge the wearable device or to leverage the potential energy reduction due to lower melt temperatures which can be translated into cycle-time reductions.
Figure 4 also highlights the need to look beyond material data sheets during the material selection stage. Teaming up with material specialists during the design phase of the project will help to optimise the design and maximise the value of the wearable.
WEARABLE DEVICES: THE NEED FOR SUPPLIER COLLABORATION
The advent of wearable injectors poses interesting and new opportunities for polymer suppliers. Product and service offerings provided by material suppliers can address this trend and contribute to a broader and faster adoption rate of wearable injectors. By diversifying and expanding its collaborations with plastic suppliers, the device development industry can leverage polymer manufacturers’ knowledge of material, conversion processes, secondary operations and predictive engineering capabilities to promote a successful launch of new medical device platforms.
REFERENCES
- “Interview: Simon Michel, Chief Executive Officer, Ypsomed”. ONdrugDelivery Magazine, June 2016 (Issue 68), pp 56–59.
- Adler M, “Challenges in the Development of Pre-filled Syringes for Biologics from a Formulation Scientist’s Point of View”. Am Pharm Rev, February 2012.
- Kanai S et al, “Reversible Self-association of a Concentrated Monoclonal Antibody Solution Mediated by Fab-Fab Interaction that Impacts Solution Viscosity”. J Pharm Sci, 2008, 97(10), pp 4219–4227.
- Cathelin J, “Through-Process Modelling for Accurate Prediction of Long Term Anisotropic Mechanics in Fibre Reinforced Thermoplastics”. 12th International Fatigue Congress, Poitier, France, 2018.
- “Critical Considerations For High Viscosity/High Volume Drug Delivery Devices”. Clinical Leader, July 2019.