Lamprou D, Mathew E, “3D-Printed Hollow Microneedles: A New Frontier For Transdermal Drug Delivery”. ONdrugDelivery, Online, February 12, 2022.
Dimitrios Lamprou and Essyrose Mathew discuss the potential of 3D-printed hollow microneedles for transdermal drug delivery.
“Microneedle drug delivery is a promising approach for transdermal drug delivery (TDD), due to its potential to deliver drug through the skin without causing pain.”
The skin is a vital organ that protects the human body from harmful substances in the environment. It is the biggest organ in the human body and has a large surface area with a rich blood supply which makes it a favourable site for transdermal drug delivery (TDD). The primary barrier between the body and environment is the uppermost layer of the skin, the stratum corneum (SC), which is around 15-20 µm in thickness and made up of a dense network of cells that gives the SC its effective barrier properties.
Microneedle (MN) drug delivery is a promising approach for transdermal drug delivery (TDD), due to its potential to deliver drug through the skin without causing pain. The micron-sized needles overcome issues of needlestick injuries and needle phobia in patients due to their small size. Hollow MNs have been of particular interest due to their ability to delivery larger volumes of drug transdermally, as well as being able to deliver drug in solution form. Hollow MNs follow a “poke and release” profile of drug release into the skin. Due to the presence of pores on the needles, hollow MNs can often be more susceptible to mechanical failure during administration, as well as blockages occurring during insertion into the skin. However, these risks can be mitigated by appropriate choice of materials and positioning of bores on the needles.
Polymeric MNs have been widely used over ceramic, metal and glass MNs due to their low cost of manufacture, availability, biocompatibility, safe disposable and ability to vary drug release profiles from the MN arrays. Micromoulding is the most commonly used method of fabrication for MN arrays, for all types of MNs including hollow MNs. Moulding techniques, especially during the initial development phase of MN designs, can introduce additional costs, as new moulds need to be created or bought each time designs are modified. Flexibility in design is essential during the research and development phase, as small changes to the geometry can regularly occur in order to modify designs. Therefore, a novel approach to the manufacture of MNs – such as additive manufacturing (AM) – can provide an array of benefits for the future of MN fabrication.
“3D printing for the fabrication of hollow MNs has had increased interest in recent years due to its fast fabrication times, scalability, accessibility and ability to explore a range of designs.”
3D PRINTING
3D printing for the fabrication of hollow MNs has had increased interest in recent years due to its fast fabrication times, scalability, accessibility and ability to explore a range of designs specific to the application – leading towards personalised medicine. During the initial research and development stage, rapid prototyping is possible as designs can be easily modified using computer-aided design (CAD) and printed directly without the need to create moulds (Figure 1).
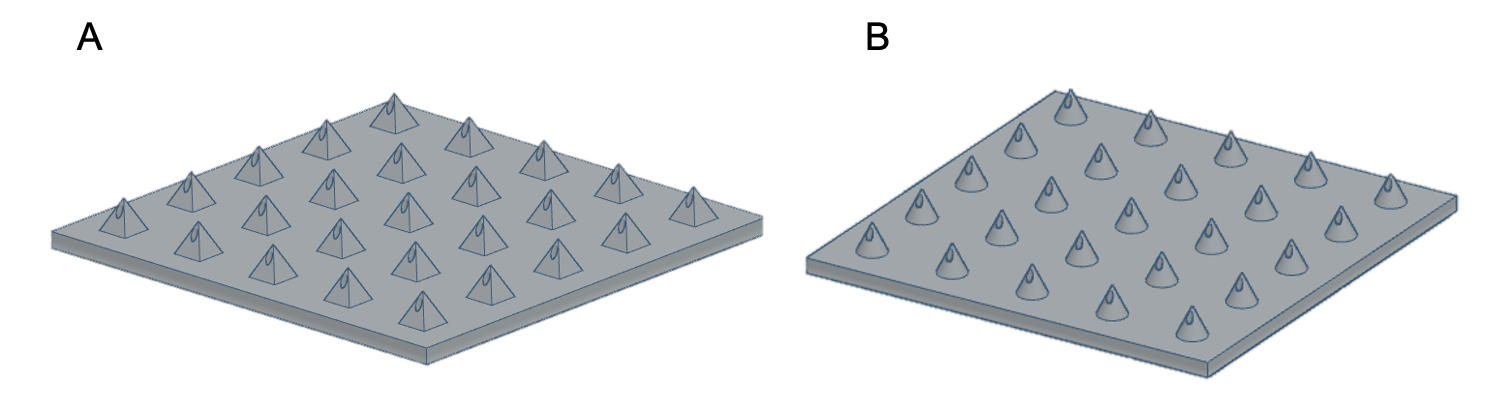
Figure 1: CAD of MN arrays.
A range of AM methods has been explored in the past for the fabrication of MN arrays, including fused deposition modelling (FDM), which is a cheap and easily accessible form of printing. However, the majority of FDM printers do not possess the resolution capabilities required for printing MNs, therefore post-printing processes such as etching are required to further sharpen the needles.
The Queen’s University Belfast (QUB) lab focuses on vat polymerisation based printing techniques for fabrication of MN arrays as they can provide printing resolutions as high as 0.01 mm. Three main types of vat polymerisation printing have been used for the fabrication of MNs, including liquid crystal display (LCD) based printing, stereolithography (SLA) and digital light processing (DLP) (Figure 2). Each of these processes offers its own advantages and disadvantages.
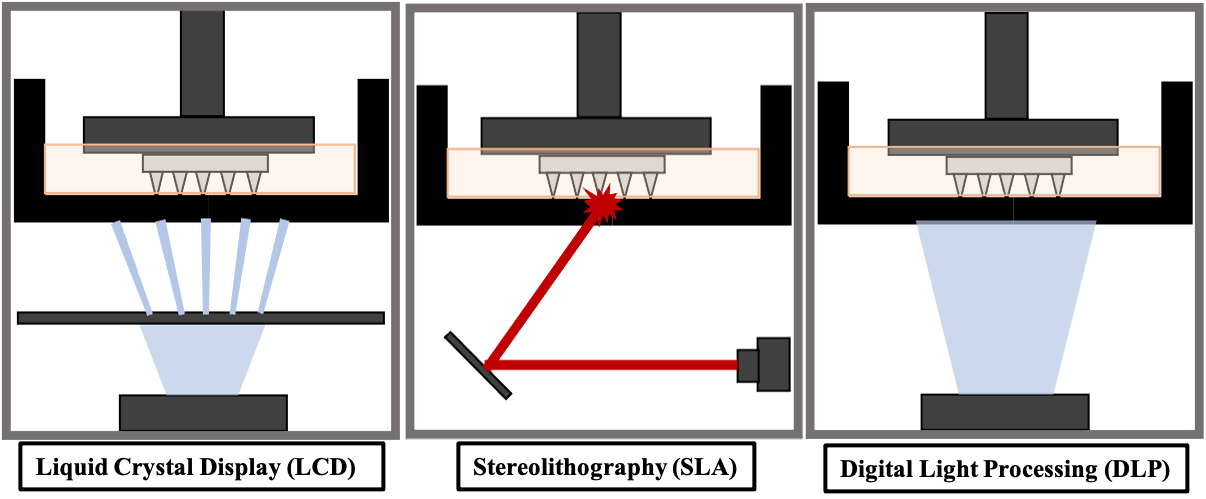
Figure 2: Resin-based 3D-printing techniques.
SLA printing is one of the most commonly used AM processes. A laser beam moves over the resin reservoir, hardening the resin at specific points throughout the resin tank, according to CAD of the print. The resin is cured in a layer-by-layer fashion, resulting in a 3D-printed object. LCD printing uses light-emitting diode (LED) light controlled by an LCD screen to project a particular pattern onto the resin VAT, where the resin is cured. Each layer of the 3D print is hardened at once and the build platform is then raised to cure the next layer of the 3D design. DLP printing uses UV light to cure the resin. Instead of an LCD screen, the projection of light is controlled by a digital light projector which flashes an image of each layer of the 3D design into the resin VAT. This process cures the entire layer at once.
LCD and DLP processes generally have faster print times than SLA printing due to the ability to cure an entire layer of the print at a time, whereas SLA is dependent on the laser beam size. LCD has the advantage of being a cheaper printer than SLA and DLP. However, DLP and SLA printing can provide higher resolutions and SLA generally produces smoother prints.
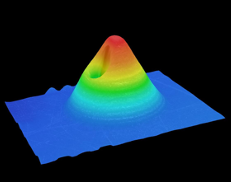
Figure 3: Digital microscope 3D reconstruction of conical MNs.
In recent studies using LCD, SLA and DLP printing, QUB found that DLP printing was able to provide optimal geometries required for producing sharp and mechanically strong MNs (Figure 3). This form of printing allows prints to be created at a high resolution and provides quicker print times compared with SLA. The printing parameters have been optimised for producing sharper needles. QUB’s research has found that the print angle plays an important role in the sharpness of the printed needles. A range of print angles was explored, along with the effect on the tip size and needle heights of MN arrays to find the optimal angle for printing.1 QUB researchers concluded that printing at an angle did make a difference to the final geometries of needles compared with printing flat to the build plate. Varying curing times were also explored to evaluate their effect on the mechanical strength of needles by evaluating the fracture of MNs using a texture analyser. By modifying some of these simple parameters when printing with resin-based printers, the final print dimensions can be optimised in order to provide sharp needles.
“MN vaccine delivery has gained increasing interest due to the potential to eliminate the need for healthcare professionals – with the opportunity for self-administration.”
FUTURE DIRECTIONS FOR TDD
In the future, the delivery of proteins and peptides using 3D-printed MN devices could be of great use. 3D-printed hollow MNs for vaccine applications is a promising area of interest. Current vaccine delivery methods can present some issues, especially with the requirement for cold storage of many drug solutions; therefore, temperature-controlled environments are needed. MN vaccine delivery has gained increasing interest due to the potential to eliminate the need for healthcare professionals – with the opportunity for self-administration. MN vaccines can also be formulated and stored in dry form, with no need for reconstitution, eliminating the need for a cold supply chain – which can be a major issue, especially for delivering vaccines to people living in warmer climates.
Another potential direction for the fabrication of MNs is the personalised curation of MN devices. There is potential for changing the shape of the MN arrangement according to the area in which delivery occurs. MNs could be fabricated according to the contours of the face or other areas of the body. The ability for MNs to fit the contours of the skin could increase the penetration efficacy of MN arrays compared with the flat base plate MNs that are currently being manufactured. This could widen the application areas for MN delivery.
“AM will be a real asset in the manufacturing of medical devices, providing a time-efficient method with potential for personalised drug delivery.”
One of the limitations for resin-based printing is the range of materials available. Photocurable resins are necessary for printing and, although there has been widening research into biocompatible resins, the choice of available materials is limited compared with conventional manufacturing techniques for MNs such as micromoulding. The regulatory considerations for 3D-printed objects to be brought to market is also high. For 3D-printed MNs to come onto the market, a detailed report is required on the manufacturing process. All chemical changes of materials must be reported in detail – changes due to photopolymerisation, as well as products of degradation. If APIs are to be combined with resins for printing, the stability of the API throughout the printing process must also be proven.
For 3D printing to become the commercial development method of MNs, there is a lot more investigation needed before it can be adopted widely. Although the US Food and Drug Administration has some “Technical Considerations for Additive Manufactured Medical Devices”, more standardised testing protocols need to be set out in order to ensure 3D-printed devices will meet regulatory requirements. Therefore, it will be some time before we will see 3D-printed MNs on the market. However, it is evident that AM will be a real asset in the manufacturing of medical devices, providing a time-efficient method with potential for personalised drug delivery.
REFERENCE
- Mathew E et al, “Optimization of Printing Parameters for Digital Light Processing 3D Printing of Hollow Microneedle Arrays.” Pharmaceutics, 2021, Vol 13 (11), 1837.
Previous article
HOW MINIATURISED LIQUID FLOW SENSORS ARE REVOLUTIONISING SUBCUTANEOUS DRUG DELIVERYNext article
PHOTO-STABILITY TESTS OF EPINEPHRINE