To Issue 132
Citation: Udale R, Eldridge C, Shah O, “Are Governments Banning the Most Environmentally Friendly Inhaler?” ONdrugDelivery, Issue 132 (Apr-May 2022), pp 30–35.
Rob Udale, Catriona Eldridge and Omar Shah assess the assertion that pMDIs are the most environmentally damaging of the available pulmonary drug delivery device archetypes, and explore some of the intricacies not accounted for in the public discourse surrounding pMDIs.
“An LCA is a methodical framework for assessing the environmental
impacts associated with a product system, from the extraction of
raw materials through to its end of life.”
INTRODUCTION
At COP26, countries committed to bold action to reduce their climate impact and achieve net zero emissions by 2050. These commitments are now percolating into the private sector, as FTSE100 companies, including AstraZeneca and GSK, announce plans to eliminate their contribution to climate change by 2050.1
Within the public sector, the UK NHS has stated its aim to be the world’s first net zero emission national health service for the emissions it controls directly by 2040 and reach an 80% reduction at some point between 2028 and 2032.2 Within this context, pressurised metered dose inhalers (pMDIs) have come under scrutiny, due to the high global warming potential of their propellants, as illustrated in a recent BBC news article.3
Some people may question the significance of asthma medication on global emissions, but data suggests that around 4% of the NHS’s entire carbon footprint comes from asthma drugs,3 and pMDIs account for 0.1% of the UK’s national carbon footprint.4 This number has been deemed too large to ignore and some parts of the NHS are looking to phase out pMDIs.5 But are dry powder inhalers (DPIs) and soft mist inhalers (SMIs) really better for the environment – and for patients – than pMDIs?
IT IS ALWAYS MORE COMPLICATED THAN THAT
Appealing though this bold conclusion is, it is over-simplistic. Firstly, it focuses only on the use phase of the device, neglecting the carbon embodied in manufacture and disposal. It also considers only the device’s CO2 equivalent (CO2e), which, although perhaps the most important, is only one of a suite of metrics used to quantify environmental impact.
To make an informed decision, a complete lifecycle assessment is required. Additionally, not all patients can safely use alternative devices (Table 1); for instance, DPIs require a sharp intake of breath that isn’t possible for some patients, particularly those with the sorts of chronic lung conditions often treated with inhaled medications.
pMDIs | DPIs | SMIs | |
Shake device before use? |
Yes | No | No |
Typical recommended intake profile |
Gentle, deep intake of air to reduce impaction of drug in the throat ▼ Hold breath for a few seconds ▼ Exhale |
Rapid deep intake of air in order to aerosolise the dose to the requisite fine particle sizes ▼ Hold breath for a few seconds ▼ Exhale |
Gentle, deep intake of air ▼ Hold breath for a few seconds ▼ Exhale |
Typical flow resistance of device |
Low | High | Medium |
Table 1: Typical use steps and required intake profile for the three inhalation device types under discussion.
LIFECYCLE ASSESSMENT
A lifecycle assessment (LCA) is a methodical framework for assessing the environmental impacts associated with a product system, from the extraction of raw materials through to its end of life. Firstly, an LCA must define the scope of the assessment: Where should it start and end the “lifecycle” in question? Is it considering the recycling and waste streams of the product?
The assessment also needs to define the functional unit. When comparing two products, it is important to make sure that the units are functionally equivalent – you can’t compare apples and oranges, but you can compare 50 g portions of different types of dried fruit. In this case, a suitable unit is one therapeutic dose from a pulmonary drug delivery system.
LCAs come in many varieties, from quick, back-of-the-envelope calculations at the concept and feasibility stage, to detailed comparative LCAs after product launch. LCAs are most valuable in the early development stages, where corrective action can be taken with relative ease, rather than waiting until a device is finished to discover its problems. LCAs can inform choices of concept, materials and even design, such as designing for disassembly.
A MORE REPRESENTATIVE ANALYSIS
To develop a more accurate picture of the environmental impact of pMDIs, they can be compared with the two currently available alternatives – DPIs and SMIs – with the functional unit as one therapeutic dose. Currently, medical devices are not widely recycled (although this is improving). To illustrate this concept, let’s perform a crude hypothetical cradle-to-grave LCA, with a “cut-off” approach (i.e. assuming no waste is recycled). For simplicity, this assessment will only consider the contribution to global warming.
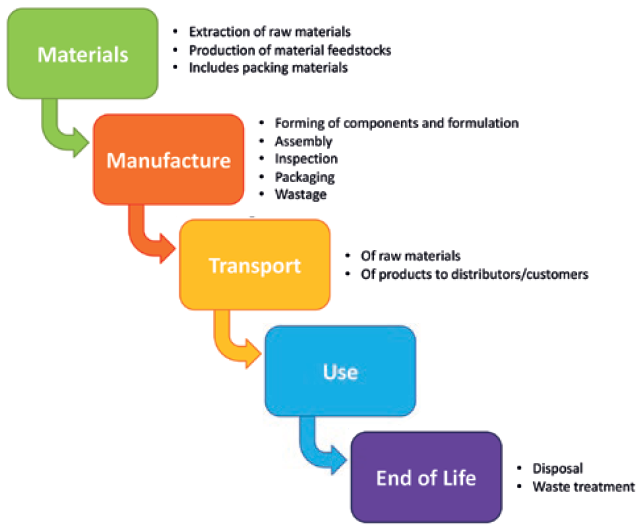
Figure 1: Stages in the life of an inhaler – or any product – at which there is the potential for emission of greenhouse gases.
The lifecycle of a device can be broken into the following stages (Figure 1):
- Materials (extraction and production)
- Manufacture (materials processing and assembly)
- Transport
- Use
- End of life.
Materials
Table 2 lists typical values of embodied carbon for different materials used in inhalers. Using such values, it is possible to estimate the CO2e engendered in the materials of these devices.
Material | pMDIs | DPIs | SMIs |
Polymers (g) | 15 | 65 | 45 |
Steel (g) | 1 | 1 | 20 |
Aluminium (g) | 6 | 8 | 2 |
Total g CO2e per device | 95 | 285 | 210 |
Number of doses | 200 | 30 | 150* |
Total g CO2e per dose | 0.5 | 9.5 | 1.5 |
Table 2: Typical masses of materials in different inhaler types. Typical embodied carbon for materials: Steel: 1.9 g CO2 g-1, Polymers: 3.5 g CO2 g-1, Aluminium: 7 g CO2 g-1 (values from references 6–8). *SMIs currently on the market are refillable a limited number of times. This figure is an estimate.
“Although DPIs and SMIs embody more carbon and send more waste to landfill, these factors are outweighed by the GWP of the propellant in pMDIs by an order of magnitude.”
Manufacturing
Without primary data on the manufacturing processes, estimating manufacturing energy cost or wastage is difficult. Additionally, these values can vary substantially by manufacturing location due to differences in the local grid energy mix. For this reason, this back-of-the-envelope LCA will neglect this phase.
Use
While DPIs and SMIs produce relatively few emissions in use, pMDIs use a hydrofluoroalkane (HFA) propellant that is released with every dose. These compounds have extremely high global warming potential (GWP) – a measure that describes how potent a greenhouse gas the compound is over a set time period, relative to CO2.
HFA-134a is the most common propellant and has a GWP of 1,300 g g-1. With a typical loading of 15 g giving 200 doses, each dose has a CO2e of 100 g. Another commonly used propellant, HFA 227ea, has a GWP of 3,350 g g-1, which is even worse.9
End of Life
Because this rough assessment is employing a cut-off approach, it assumes none of the materials are recycled; therefore, the only salient figure of merit is the mass of material sent to landfill, shown in Table 3.
Inhaler type | pMDIs | DPIs | SMIs |
Typical total mass (g) | 22 | 75 | 65 |
Mass per dose (g) | 0.1 | 2.5 | 0.4 |
Table 3: Typical masses of different inhaler types.
Inhaler type | HFA-134a pMDI | DPI | SMI |
CO2e per dose engendered in the materials (g) | 0.5 | 9.5 | 1.5 |
CO2e per dose emitted during use (g) | 100 | 0 | 0 |
Total CO2e per dose (g) | 100 | 9.5 | 1.5 |
Table 4: Total equivalent CO2 per dose, including materials and usage.
Summary
What this assessment shows is that, although DPIs and SMIs embody more carbon and send more waste to landfill, these factors are outweighed by the GWP of the propellant in pMDIs by an order of magnitude (Table 4). This highlights the need for improvement in this area, which could potentially make pMDIs the green choice compared with the competition.
Another factor to consider is the relative complexity of DPIs and SMIs. pMDIs are much simpler, which not only makes them cheaper to produce, but also easier to design for recyclability, giving them greater potential for improvement.
DESIGNING BETTER DEVICES
This simplistic LCA shows that the propellant is the root cause of the majority of a pMDI’s carbon footprint – a conclusion with which the Carbon Trust’s (London, UK) analysis of GSK’s inhalers agrees. Approximately 75% of GSK’s Seretide MDI’s carbon footprint comes from the HFC-134a propellant.10 To combat this, the inhalation sector and pMDIs are moving rapidly towards lower impact propellants with isobutane, HFA-152-a from Koura11 (MA, US) and Honeywell’s (NC, US) HFO-1234-ze(E)12 currently leading the race to replace existing propellants.
Adopting these replacements will require a significant redesign of the standard pMDI canister and valve components, as well as adapting the filling process, due to 1234ze and 152a’s flammability. However, this change will reduce the GWP of pMDIs by an order of magnitude.9 This could bring pMDIs into line with, or even ahead of, the competition. But what other changes can improve a product’s sustainability? And when can they be made?
At Product Launch
Once a product reaches the market, the possible reductions are limited. Recycling schemes, like Chiesi’s TakeAIR13 or GSK’s Complete The Cycle (now defunct),14 do exist, and a good understanding of an inhaler’s sustainability should include recyclability. An estimated 0.5 million tonnes of CO2e would be saved annually if every inhaler in the UK was recycled.15
Recycling does some good – for example, unused propellant can be used in air conditioning units and aluminium cans can be reclaimed – but it is limited. Without infrastructure for separate waste collection, there will be no recycling at all; GSK’s recycling scheme achieved a <1% collection rate. Even with collection schemes in place, most of the plastic components will still go to landfill or energy-from-waste schemes.14 Furthermore, recycling cannot reverse environmental impacts that have already occurred; it cannot recapture propellant which has already been released during use, or un-spend the energy used to make the device. However, better design can reduce or remove these impacts before they occur. In comparison, recycling is, at best, only mitigation, not a cure.
Designing for Manufacture
Larger reductions are possible earlier in product development. Stepping back just to selecting manufacturers, careful choices can reduce environmental impact. Selecting manufacturers with more renewable energy supplies reduces CO2e emissions, and reducing the distance between manufacturer and customer reduces transport emissions. An inhaler manufactured in Germany would be produced using approximately 50% renewable energy,16 but then would have to travel a significant distance to reach a customer in the US, and it would cost considerably more than a product manufactured in China (Figure 2).
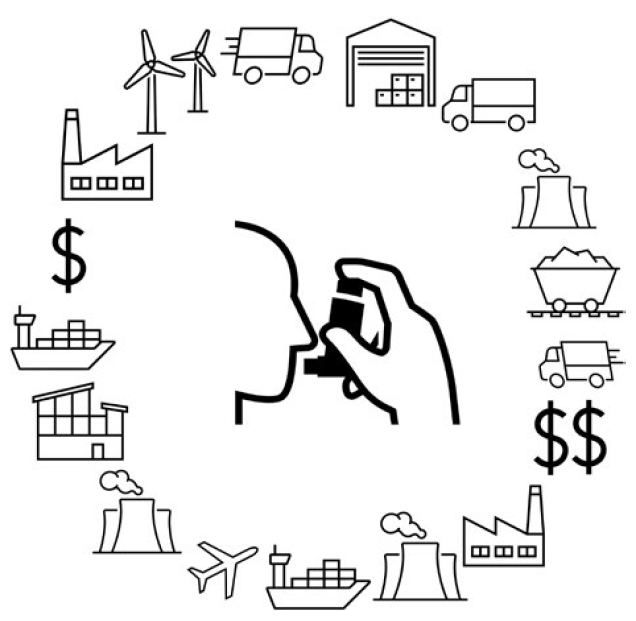
Figure 2: Illustration of the trade-offs manufacturers face when choosing the manufacturing location.
Additionally, manufacturing methods will affect the device’s environmental impact – for example, efficient manufacturing equipment, such as electric rather than hydraulic plastic injection moulding machines, could reduce energy costs by over 30%,17 and vacuum-crimping the canister rather than purging with propellant could also make significant reductions. All these considerations should be taken into account when assessing sustainability; LCA is a useful framework for balancing these trade-offs.
“Such assessments demonstrate that the environmental impact of current pMDIs is not significantly different from that of their competitors and, with the development and introduction of new, lower-GWP propellants on the horizon, they have the potential to become better.”
During the Design Phase
To achieve more fundamental improvements, it is prudent to consider the design itself. Here, material choices and more efficient design can reduce the environmental impact of a device; for example, selecting and designing for a lower-impact propellant, choosing steel over aluminium or titanium, or designing for efficient manufacturing methods, such as injection moulding, rather than more wasteful ones, such as milling.
Recyclability can also be designed in at this stage, such as designing for a more easily disassembled device and implementing single-polymer components. With ease-of-separation designed in, more of the device can actually be recycled at end of life, rather than going into landfill or energy-from-waste schemes. There are now collection-for-recycling schemes for items ranging from lithium batteries18 to injector pens,19 as well as material-specific schemes, including medical PVC pilot schemes.20 Medical-grade materials have great recycling potential, due to their high quality and traceability. However, if components cannot be separated into different materials, they often cannot be usefully recycled.21 Without designing-in recyclability, this high-value waste is lost to landfill.
Counterintuitively, designing away from recyclability can reduce overall environmental impact; a less recyclable device with a longer working lifetime may have a lower impact overall than a fully recyclable but shorter-lived alternative. For example, a reusable core with disposable sub-systems could minimise both environmental impact and cost. This approach is extremely pertinent in the development of connected devices, where the material and environmental costs of the electronics sub-system are large compared with the rest of the device. LCA provides a framework for comparing the impact of a product’s embodied energy, lifetime, function and end of life.
The Concept Stage
The biggest reductions are possible at the concept stage. Most pMDI emissions are from the propellant. At the concept stage, a design can switch between propellants, or from a single use to a partially reusable device, with relative ease, potentially significantly lowering the device’s overall environmental impact. Such changes would be significantly harder later in the design process.
A simple, small-scale LCA in the early design stages can help companies identify their most (and least) sustainable options and avoid locking in an inherently unsustainable design. If sustainability is ignored until the product launches, it will be too late to improve a product’s fundamental sustainability without expensive redesigns and manufacturing adjustments.
Designing for sustainability can be counterintuitive; direct comparisons of products in use can miss the wider picture and can artificially inflate or obscure the impact of various design choices. A full-lifecycle approach to assessing sustainability is vital for good design and is most effective when it is part of the design process from the start.
“The NHS and other payers should not only look at emissions during use, but rather assess the full lifecycle before deciding to switch from one type of device to another.”
CONCLUSION
The NHS and other payers should not only look at emissions during use, but rather assess the full lifecycle before deciding to switch from one type of device to another. Such assessments demonstrate that the environmental impact of current pMDIs is not significantly different from that of their competitors and, with the development and introduction of new, lower-GWP propellants on the horizon, they have the potential to become better.
However, this drive for reduced environmental footprint must be balanced with the needs of the other stakeholders – principally, cost to the payer and outcomes for both payer and patient – and balanced against the strengths and weaknesses of alternative devices (Table 5). Historically, the key advantages of pMDIs have been their suitability for children and patients with breathing limitations, and their low cost – will that remain the case with the new propellants?
pMDIs | DPIs | SMIs | ||
Simple device (no breath actuation) |
Breath-actuated device | |||
Example | GSK Ventolin, Kindeva Sirdupla |
Kindeva Autohaler, Mundipharma K-haler, Teva Easi-breath |
GSK Elipta, Novartis Breezhaler, Chiesi NEXThaler | Boehringer Ingelheim’s Respimat |
Environmental impact | ↑↑ | ↑↑↑ | ↑ | ↑ |
Cost per dose | $ | $$ | $$ | $$$ |
Delivery efficiency |
Low to Moderate Require co-ordination between inhalation and actuation, leading to variable delivery efficiency drug delivery |
Moderate Inhalation and activation controlled so more consistent delivery efficiency |
Moderate |
Best in class22 Excellent fine particle fraction (FPF) and easy to co-ordinate, hence high delivered dose |
Advantages | Small and discreate Commonly hold a large number of delivered doses Total isolation of the drug from the external environment |
Highest mass of drug per dose of all the inhaler forms Dose counters included |
Small and portable Require less breath co-ordination than pMDIs, due to low soft mist velocity, and less of a sharp breath than DPIs |
|
Disadvantages | Require shaking and priming – which is often forgotten about, leading to variable dose efficiency Developing physically and chemically stable formulations compatible with HFA propellants is challenging |
The sharp intake of breath that is commonly required isn’t possible for all patients Difficulty in developing a stable powder formulation that meets the challenging requirements for good aerosol performance |
Relatively low number of doses per device Not yet suitable for all drug formulations and drug needs to be resistant to high shear forces in nozzle |
Table 5: Illustration of the typical strengths and weaknesses of the three main inhaler types.22
By employing good design principles and LCAs throughout the design process, it is possible to meet the requirements of both the end user and the wider stakeholders. Watch this space – pMDIs may well be the green option in 10 years and should not be banned!
REFERENCES
- “COP26 Sees UK Businesses Lead the World in Climate Change Commitments”. Press Release, UK Government, Nov 4, 2021.
- “Delivering a ‘Net Zero’ National Health Service”. UK NHS, Oct 2020.
- Gallagher J, “Why Switching Asthma Inhaler Could Be Better for You and the Planet”. BBC News, Feb 2022.
- Keeley D, Scullion JE, Usmani OS, “Minimising the Environmental Impact of Inhaled Therapies: Problems with Policy on Low Carbon Inhalers”. Eur Respir J, May 2020, Vol 55(5), Article 2001122.
- “Primary Care Networks – Plans for 2021/22 and 2022/23: Annex B – Investment and Impact Fund (IIF): 2021/22 and 2022/23”. UK NHS, Oct 2021.
- “Climate Change and the Production of Iron and Steel”. World Steel Association, May 2021.
- “Process Data Sets”. Plastics Europe Public LCI Database, accessed Mar 2022.
- “Fact Sheet: Carbon Footprint of Aluminium”. Company Web Page, Alupro, accessed Mar 2022.
- Noakes T, Corr S, “Metered Dose Inhaler Propellants”. Mexichem, Dec 2016.
- “GlaxoSmithKline – Value Chain Footprint”. Company Web Page, Carbon Trust, accessed Mar 2022.
- “A Q&A about HFA 152a with Koura’s Simon Gardner”. OINDP News, Oct 2021.
- “AstraZeneca’s ‘Ambition Zero Carbon’ Strategy to Eliminate Emissions by 2025 and be Carbon Negative Across the Entire Value Chain by 2030”. Press Release, AstraZeneca, Jan 22, 2020.
- “Chiesi Group Calls for #ActionOverWords in the Fight Against Climate Change”. Press Release, Chiesi, May 10, 2021.
- “GlaxoSmithKline ‘Complete the Cycle’ Inhaler Recycling and Recovery Scheme Now Accessible UK-Wide”. Nursing Times, Feb 2013.
- “Inhalers”. Web Page, Recycle Now, accessed Mar 2022.
- “Germany’s Power Mix 2020 – Data, Charts & Key Findings”. Strom-Report, July 2021.
- Thiriez A, Gutowski T, “An Environmental Analysis of Injection Molding”. Massachusetts Institute of Technology graduate thesis, 2006.
- “Give Your Used Batteries a New Life”. Web Page, Bebat, accessed Mar 2022.
- “PenCycle”. Novo Nordisk, Oct 2021.
- “RecoMed”. Web Page, RecoMed, accessed Mar 2022.
- Ross K, “The Effective Reuse and Applications for Mixed Plastic Waste”. Web Page, British Plastics Federation, accessed Mar 2022.
- Kunkel G et al, “Respimat (A New Soft Mist Inhaler) Delivering Fenoterol Plus Ipratropium Bromide Provides Equivalent Bronchodilation at Half The Cumulative Dose Compared with a Conventional Metered Dose Inhaler in Asthmatic Patients”. Respiration, 2000, Vol 67(3), pp 306–314.