To Issue 145
Citation: Churro R, Lopes C, Pires J, “Capsule Filling for DPIs – Linking Process Scales Through Advanced Characterisation and Quality by Design”. ONdrugDelivery, Issue 145 (Apr 2023), pp 34–38.
Rui Churro, Carolina Lopes and João Pires discuss the value of rheological characterisation of powders to expedite and de-risk the scale-up of DPI capsule-filling processes.
INTRODUCTION
Dry powder inhalers (DPIs) are becoming increasingly popular to treat both respiratory and systemic diseases as they are able to achieve better physical and chemical stability of the drug product. Conventional DPI formulations are traditionally composed of physical mixtures of coarse carriers with one or more micronised APIs, typically with an aerodynamic particle size below 5 μm.1 While a well-established formulation strategy, carrier-based drug products come with several drawbacks, spanning from difficulties in uniformity of delivered dose to inefficient drug deposition.
“The wide adoption of capsules comes from them being a cost- effective solution with proven drug stability, as well as from being able to leverage well-established capsule-filling technologies and capacity.”
Composite particles are, therefore, receiving increased attention as an alternative formulation strategy for DPIs. In these formulations, the API is embedded into an excipient matrix, in single engineered particles with low densities and small aerodynamic particle sizes that can be efficiently delivered to the lung. However, these unique properties can also cause operational challenges while processing these powders. Due to their large surface area and low densities, composite powders can be highly cohesive, resulting in poor flowability and intense aggregation during the downstream filling processes.
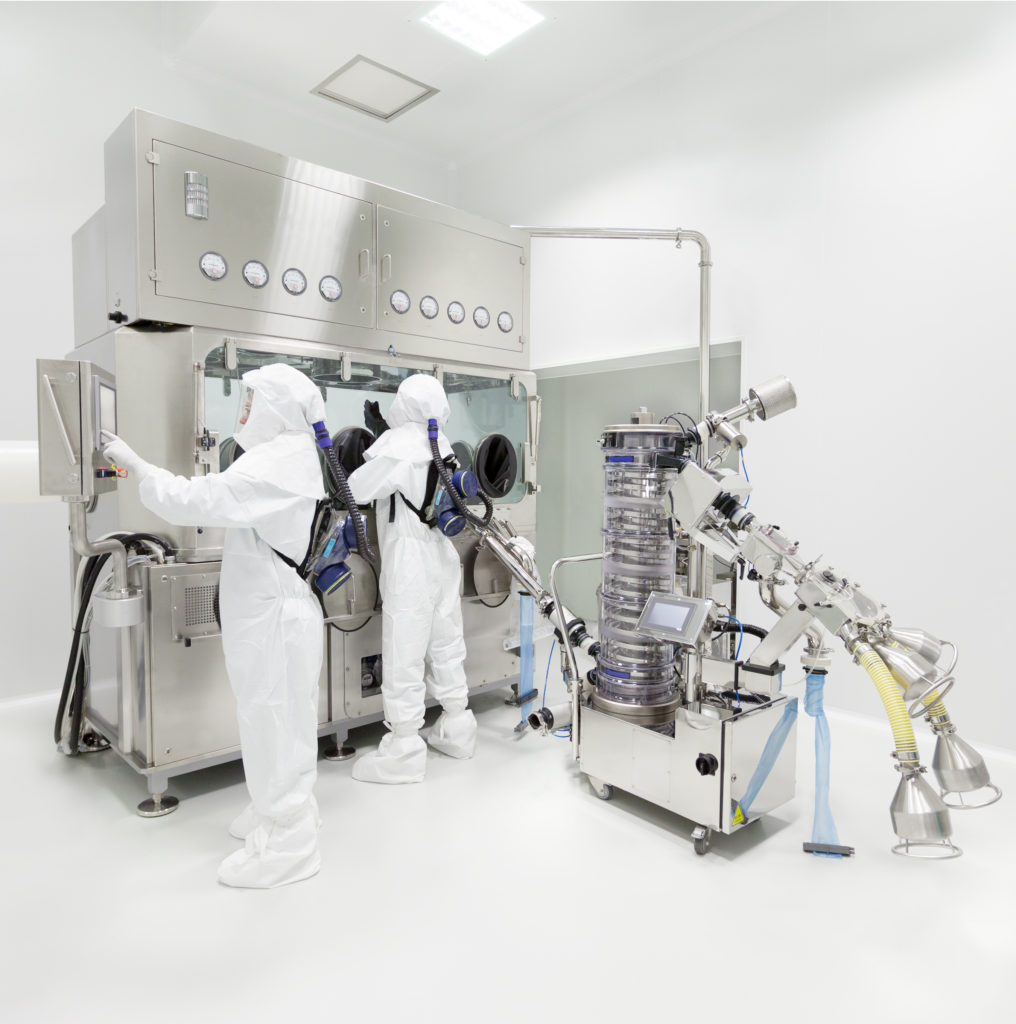
Figure 1: Large-scale capsule filling process at one of Hovione’s manufacturing lines.
In DPIs, capsules are widely used as reservoirs for the formulation to be later inserted into a device that is then actuated by the patient for self-administration. The wide adoption of capsules comes from them being a cost-effective solution with proven drug stability, as well as from being able to leverage well-established capsule-filling technologies and capacity (Figure 1). To fill these capsules, several manufacturing technologies are commercially available, with dosator-based and vacuum-drum filling being two of the most popular types for low-dosage solutions.
On the one hand, dosator-based capsule filling is already a well-established technology for DPIs in the pharmaceutical industry. Several studies can be found addressing material property dependency and process parameters, especially for carrier-based formulations. However, the same does not apply to composite formulations, with little information available on the performance of dosator-based technology using highly cohesive and poorly flowable powders.
On the other hand, vacuum-drum filling has also been identified as an accurate filling solution for capsule-based DPIs and is currently gaining traction in the industry. Few case studies are still available regarding its application for DPI formulations, although its working principles are thought to promote a more accurate filling process, especially for low-dosage composite formulations.2
The selection of the best filling technology and process conditions is critical, as DPI formulations may contain doses as low as a few milligrams. Indeed, for dosages as low as 5 mg, a single milligram difference can determine the acceptance or rejection of a filled capsule. Therefore, to achieve such a high level of accuracy and select the most suitable process parameters, it is essential to understand the filling powder’s flowability at conditions representative of the manufacturing processes.2
Indeed, the characterisation of the rheological properties of inhalation-based powders can provide valuable insights for both formulation and process optimisation, as one tries to achieve a specific capsule fill weight and aerodynamic performance. For capsule filling, especially for low dosages, powder compressibility, density, cohesiveness and, ultimately, flowability can be derived from analytical characterisation techniques. However, limited information is available regarding the relationship between rheological properties and the operational space of potential critical process parameters, such as vacuum pressure in drum-filling processes, among others.
Due to the advantages of composite formulations for DPIs, but also due to their inherent challenges, Hovione has been deeply investing in developing formulation and process development strategies together with advanced characterisation tools to anticipate operational and performance risks from the very early stages of development. In this way, the company aims to minimise the development effort at both lab and manufacturing scales. In the following sections, a set of different case studies is presented, showing how such strategies can support capsule-filling process development with different technologies and at multiple scales.
DEFINING THE OPERATIONAL SPACE OF DIFFERENT FORMULATIONS
“At Hovione, composite systems strategies are already an established platform for the formulation development of DPIs.”
At Hovione, composite systems strategies are already an established platform for the formulation development of DPIs. As mentioned prior, formulations outside the carrier-based space typically exhibit poorer flowability because of their higher cohesiveness. Moreover, depending on the formulation – API alone versus spray-dried composite (considering different excipients), for example – the rheologic behaviour can vary strongly and, therefore, impact the conditions that should be used in downstream processes, such as capsule filling.
Overlooking the flow properties of a given formulation can potentially prevent the identification of operational problems, such as inconsistent dosing, erratic flow and even complete blockages in the capsule filler equipment, which can affect manufacturing robustness and drug product quality. Therefore, understanding how these powder properties influence the filling process is essential to optimise the process parameters and achieve the desired outputs.
Shear cell testing is a widely used method for evaluating the flow properties and powder behaviour of different materials, providing valuable insights into the performance and quality of powders in several applications. For DPIs, such information can be used to guide both formulation and process development towards the optimisation of the filling process and the powder’s aerodynamic performance.
For example, the comparison between the fundamental rheological properties of a micronised crystalline API alone and a composite formulation composed of 80%:20% w/w trehalose dihydrate and L-leucine was determined using the yield locus test (Table 1). Hydroxypropyl methylcellulose (HPMC) size 3 capsules were later filled with these formulations using a lab-scale drum filler (Drum Lab – Harro Höfliger) to correlate the measured rheologic properties with the performance of the filling process.
Formulation | d(0.1) (μm) | d(0.5) (μm) | d(0.9) (μm) | FFC (-) | FFρ (-) | φE (º) | T,C (Pa) |
Composite | 0.6 | 2.9 | 6.8 | 1.3 | 0.5 | 62.3 | 838 |
Micronised | 1.1 | 2.6 | 5.3 | 2.3 | 0.8 | 45.9 | 444 |
FFC – Flow function coefficient; FFρ – Flow function coefficient normalised for bulk density; φE – Effective angle of internal friction; T,C – Cohesion
Table 1: Particle size and shear cell powder characterisation at constant vertical normal stress at pre-shear.
Both formulations showed different flow properties, indicating that the composite formulation (flow function coefficient (FFC) of 1.3) was more cohesive than its API-alone counterpart (FFC of 2.3). As seen in Figure 2, this prediction was later confirmed during the capsule-filling process as, for the same target fill weight, higher vacuum pressure was required for the API-alone formulation. Furthermore, the API-alone formulation also had a tighter vacuum pressure range to achieve the same target 10 mg fill weight. Together, these outcomes indicate a clear dependence of the operational ranges on the type of formulation, supporting the potential value of rheological results in defining adequate process parameters, values or ranges, even before performing any experiments.
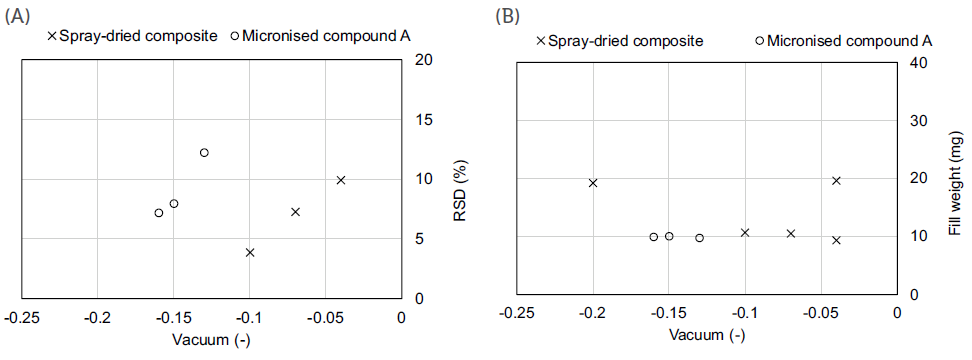
Figure 2: Vacuum drum filling process performance (A) and operational space (B).
SCALING UP A COMPOSITE FORMULATION WITH DRUM FILLING
Once the flowability properties of a formulation are known, the next step is to focus on developing the capsule-filling process at lab scale, with the goal of successfully transferring the process to manufacturing scale. A possible example would be developing a capsule-filling process for the composite formulation used in the previous section at lab scale using a Drum Lab in order to de-risk a later scale-up to a pilot scale. In other words, this translates into scaling up a process from a throughput as low as six doses per minute up to 25,000 caps per hour, while assuring identical product quality.
In that regard, Hovione supports drug product development under quality-by-design (QbD) methodologies, namely with the early identification of potential critical process parameters (pCPPs) and potential critical quality attributes (pCQAs). In the case of drum filling for capsule-based DPIs, powder fluidisation, vacuum pressure for plug formation and stirrer offset are factors often selected as pCPPs, while aerodynamic performance, uniformity of delivery dose and fine particle dose are some of the most common pCQAs. Additionally, other important indicators, such as relative weight variability and capsule rejection rate or yield, are also considered for optimising process performance and robustness.
For this specific case study,3 as seen in Figure 3, lab-scale trials indicated that higher vacuum pressures and sieving of the feed material are the most important contributors for process robustness. Conversely, the effect of the stirrer offset was found to be negligible for the chosen formulation.
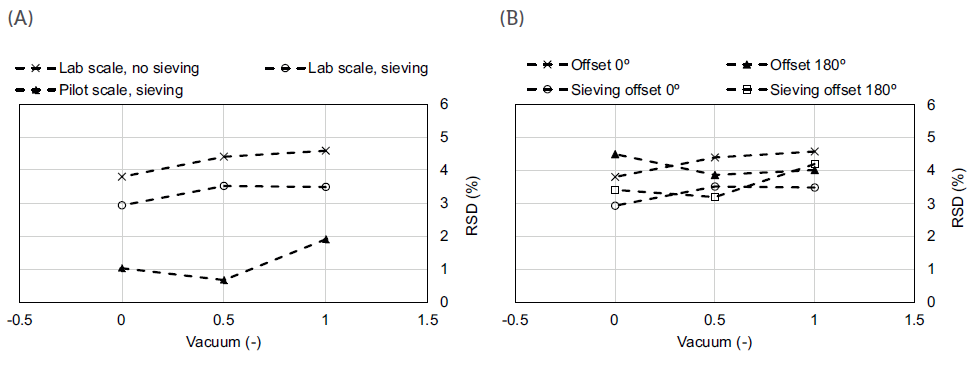
Figure 3: Capsule-filling process performance for the sieving and scale tests (A) and for the sieving and offset tests at the lab scale (B).
“Once the flowability properties of a formulation are known, the next step is to focus on developing the capsule-filling process at lab scale, with the goal of successfully transferring the process to manufacturing scale.”
Regarding vacuum pressures, process robustness improved with increasing vacuum pressure, which can be attributed to the formation of more compacted plugs, which can eventually result in lower fill-weight variability. However, care should be taken while pursuing higher vacuum pressures, as overly compacted material may prevent the redispersion of the plug and compromise the efficiency of powder aerosolisation in the DPI and subsequent delivery to the lung. Regarding the sieving step, capsules with sieved material at lab scale presented an improved aerodynamic performance. However, this processing step might well have contributed to increased powder cohesiveness, resulting in a slightly lower emitted dose observed for these capsules.
Having these results in mind, a similar process analysis was later performed at the pilot scale.3 The results confirmed a similar response in filling performance to differences in vacuum pressure at both lab and pilot scale. However, it can be concluded that lab-scale trials always performed worse than the ones performed in the pilot unit – a higher variability was always obtained in the former for the same process conditions.
Both set of results indicate that lab-scale trials can be used as a process development and de-risking tool of the process at scale because:
- Impact of process parameters on process performance presents similar trends for both scales;
- The process developed at lab scale represents a worst-case scenario in comparison with the pilot scale.
Using this strategy, Hovione’s QbD approach to process development of inhalation drug products can significantly reduce risk during scale-up, while also adding robustness and predictability during routine supply.
“Hovione equally supports dosator-based capsule-filling process development through a QbD approach, going from a manual dosator at lab scale to either pilot scale or to a fully commercial scale.”
SCALING UP A COMPOSITE FORMULATION WITH DOSATOR FILLING
Hovione equally supports dosator-based capsule-filling process development through a QbD approach, going from a manual dosator (MG2) at lab scale to either pilot scale (MG2 FlexaLab, up to 3,000 caps/h) or to a fully commercial scale (MG2 TEKNA, up to 55,000 caps/h). All this can be achieved by using the same dosators across scales through higher filling speed and multiple dosators, thereby facilitating progressive scale-ups, reducing development effort and minimising potential risks in product quality and operations.
Similar to the drum-filling process, a risk-assessment-based approach to the definition of an operational space is also employed from the early development stages. In dosator-based capsule filling, process parameters such as compaction ratio, filling speed and environmental conditions are typically considered pCPPs, alongside the same pCQAs and process indicators described for vacuum-drum processes.
As an example, a similar composite formulation from the previous sections was filled at a pilot scale (FlexaLab, MG2).4 Capsules were filled with three different fill weights (5, 10 and 20 mg), using different dosator diameters, powder-bed heights and filling speeds (Table 2). For the 20 mg capsules, rejection rates were very low across the different levels of compaction tested, indicating a wide operational range at the pilot scale. However, as lower fill weights were attempted, higher rejection rates were obtained due to increased levels of compaction.
Average Weight (mg) | Dosator Diameter (mm) | Compaction Ratio (-) | Rejection Rate (%) | ED (%) | FPF (ED %) |
20.9 ± 0.7 | 2.8 | 0.9 | 0 | 97.4 | 57.0 |
20.1 ± 0.6 | 3.4 | 0.7 | 0 | 103.1 | 58.5 |
20.0 ± 0.4 | 3.7 | 0.5 | 3 | 96.7 | 69.3 |
10.7 ± 0.0 | 2.8 | 0.6 | 20 | – | |
9.7 ± 0.3 | 2.8 | 05 | 0 | 88.7 | 72.7 |
4.9 ± 0.8 | 2.2 | 0.5 | 18 | – | |
5.1 ± 0.3 | 1.9 | 0.8 | 0 | 83.1 | 80.1 |
20 | 3.7 | (Hand filled) | – | – | 67.0 |
FPF – Fine particle fraction; ED – Emitted dose
Table 2: Dosator filling process parameters and performance characterisation.
Consequently, the optimal chamber/layer compaction ratio values decreased with the target fill weight, although all were below a ratio of one, which would theoretically mean no pre-compression of the powder. Such trends come from the fact that the smaller dosators used for lower fill weight require a lower compaction ratio to transfer the formed plug from the powder bed into the capsule. Contrary to compaction ratio, capsule-filling speed had no significant impact on capsule-compaction level or rejection rate. Finally, similar aerodynamic performances were obtained at both lab and pilot scales.4 Once more, these results indicate a good match between early development studies, with small material needs, and the output from the process at later stages and higher throughputs.
CONCLUSION
Overall, although highly promising for DPIs, composite formulations bring multiple challenges to development, especially during the downstream capsule filling process. However, the case studies presented here show that, through advanced characterisation tools and QbD-based process development, it is possible to anticipate challenges from the early stages and act upon them through formulation and process optimisation. This approach allows Hovione to accelerate the transfer of capsule-filling processes to larger scales, for both clinical and commercial supply, by minimising the required development effort and the level of risk associated with each scale-up.
REFERENCES
- Cordts E, Steckel H, “Formulation considerations for dry powder inhalers”. Ther Deliv, 2014, Vol 5(6), pp 675–689.
- Jüptner A, Scherließ R, “Spray Dried Formulations for Inhalation – Meaningful Characterisation of Powder Properties”. Pharmaceutics, 2019, Vol 12(1), p 14.
- Churro R et al, “Capsule Filling of Spray Dried Powders for Inhalation using a Drum Filling technology: From Lab to Pilot Scale”. Poster presentation at DDL, 2022.
- Braga M et al, “Spray dried composite powders: capsule filling process optimization and aerodynamic performance characterization”. Poster presentation at DDL, 2018.