Citation: Seaward D, Phasey D, “Drivers for Change in Aseptic Automation”, ONdrugDelivery, Issue 120 (May 2021), pp 72–77.
Dave Seaward and David Phasey discuss the factors that have led to recent growth in aseptic automation within the pharmaceutical industry.
“A rise in insoluble small molecules and biologics [is] driving more parenteral applications. In turn, this is leading to growth in aseptic automation dominated by fill-finish.”
MACRO TRENDS
The last few years have seen a significant shift in focus by aseptic machinery vendors as they respond to their clients’ demands. This is merely a reflection of structural changes within the pharmaceutical industry. Parenteral drug delivery remains an area of significant growth, with the trend towards home healthcare and self-administration of injectables (to reduce administration costs and improve the “patient journey”).
Overlaid upon this, is a rise in insoluble small molecules and biologics driving more parenteral applications. In turn, this is leading to growth in aseptic automation dominated by fill-finish.
The rise of biologics and, more recently, some of the cell and gene therapies – also known as advanced therapy medicinal products (ATMPs) – has driven much of this change within aseptic machinery vendors. Manufacturing facilities are having to adapt to ever-smaller batch commercial manufacturing. Vendors are developing flexible automation systems to serve the growth of biologics, orphan drug products and personalised medicines.
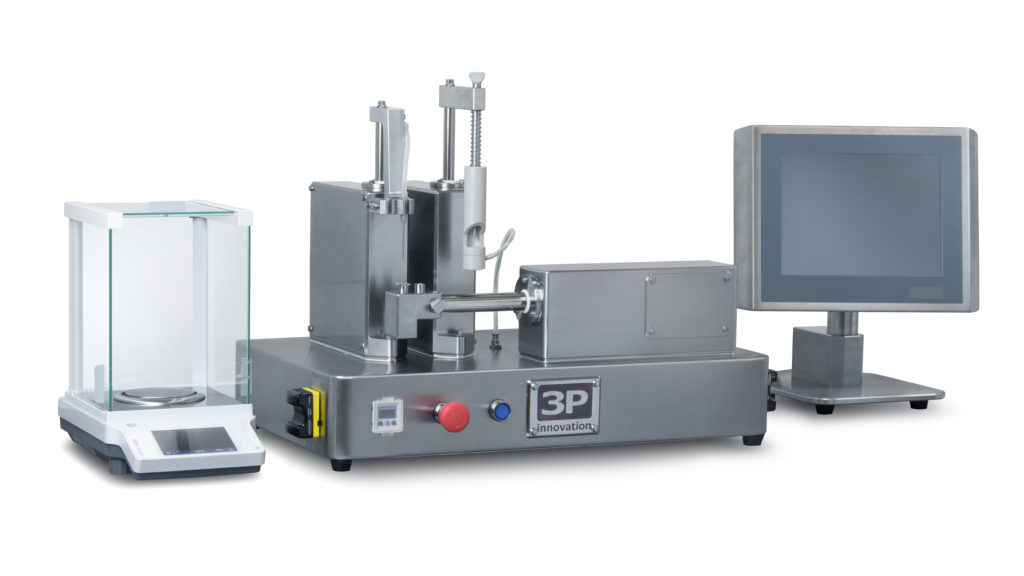
Figure 1: Ultra-low scale fill-finish (3P’s F2V).
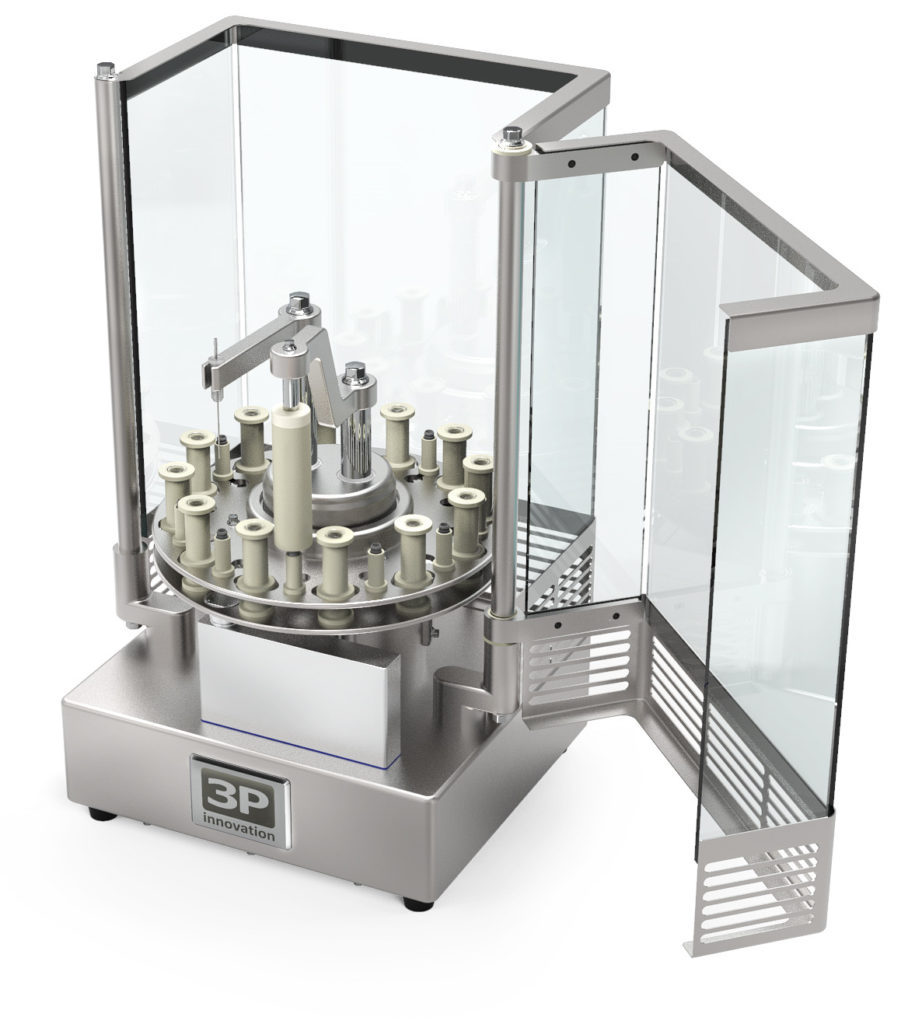
Figure 2: Low-scale fill-finish (3P’s F2V-W-N-S).
This has led to the need for flexible machinery capable of smaller batch sizes (see Figures 1 and 2), the rise of robotics and the need for custom machinery to assemble novel administration devices. While some products still require high-speed, high-volume and relatively inflexible fill-finish machinery, vendors are increasingly offering smaller footprint flexible machines filled with reconfigurable robotic processing.
The borosilicate glass vial remains the go-to primary drug container for many of these medicaments, especially during clinical trials and initial commercial manufacture. There is, however, a continued trend towards prefilled syringes (PFSs) and cartridges that typically are then used within autoinjectors, dual chamber/reconstitution applications and wearable devices. This trend can be traced back to the early 1980s, when Sanofi and Rhône Poulenc- Rorer (now Sanofi-Aventis) successfully introduced them as the primary drug container for heparins. Prior to this, PFSs were very much a niche market product.
The use of plastics and, in particular, cyclic olefin copolymer (COC), is opening up many new primary drug container opportunities – this material can enable the elimination of silicone oil lubricants that interact with some drugs (~10% of the biologic pipeline drugs are ultra-sensitive to silicone), it is more robust at cryogenic temperatures, and injection moulding provides for the addition of features to be moulded onto the primary drug container. The ability to add features via injection moulding to the primary drug container enables the medical device designer to get really creative – in particular, this is leading to more compact devices.
The trend towards PFSs from vials is likely to continue as they enable easier and safer injections. Since the drug is already filled in the container in its exact dose, this prevents the risk of dosing error and reduces overfill during the production of a potentially very costly drug.
“Industrial robotics are seeing a boom across many industrial sectors. This is driven by a need for improved product quality, greater assembly precision and greater automation flexibility. In previous decades, the cost of robots often precluded their implementation. However, ever lower cost robots can now lead to very rapid paybacks.”
Many of these drugs are high value and low volume. As such, the space and capital equipment cost required to depyrogenate/ sterilise primary drug containers ahead of a fill-finish line is falling out of favour for all but the largest volume production facilities. In its place are the use of presterilised tubs containing nests of primary drug containers (according to ISO11040-7:2015[en]). This has led to smaller fill-finish production equipment focused on the rapid sanitisation of the outside of the tubs prior to removal of the Tyvek™ (DuPont, Wilmington, DE, US) lids and the fill-finish process. Robots are the natural solution to de-nesting (and re-nesting) tubs of primary drug containers. Simple changes to the robot end effector also enable the same line to process vials, cartridges, PFSs and novel primary drug containers when packages are in ISOstandard tubs. To minimise development, many vendors of aseptic automation have elected to use “standard” robots, albeit to cleanroom/sterilisable standards (3P uses a Stäubli (Freienbach, Switzerland) integrator). Typically, these six-axis or SCARA robots are relatively large for the application, leading to larger-than necessary isolators. More recently, vendors have begun to introduce their own compact robots (3P has recently introduced the “Crabot” tub-handling robot).
Industrial robotics are seeing a boom across many industrial sectors. This is driven by a need for improved product quality, greater assembly precision and greater automation flexibility. In previous decades, the cost of robots often precluded their implementation. However, ever lower cost robots can now lead to very rapid paybacks. Notwithstanding the desire for more consistent process aseptic processing, robots enable a reduction in particulates. Numerous articles have demonstrated that human operators are the main contamination risk in cleanrooms, particularly through the shedding of particles from personal clothing (even undergarments) and skin, exacerbated by movement. A typical person sheds around one billion skin cells every day and 10% of them have microorganisms on them.1 Meanwhile, every minute, microorganism-loaded liquid droplets are released from the mouth and nose (10 million/g of saliva or nasal fluid).1 Hence, manual aseptic tasks are increasingly being converted to non shedding robotic tasks typically within an isolator.2 To paraphrase another machinery company, “if it can be automated, it will be automated!”
“In connection with the trend towards COC, there are a number of novel devices in development taking advantage of the flexibility of injection mouldings to create patient benefits. Some of these unique features are driving the need for custom automation and custom fill-finish solutions.”
THE TREND TOWARDS CUSTOM AUTOMATION
The previous paragraphs refer to the macro trends that all aseptic automation companies are observing. As experts in custom aseptic automation, 3P has some unique insights into many of the niche applications – in many cases, these have been under development for several years and they are now starting to appear on the market. These applications require assembly and fill-finish equipment that simply did not exist before 3P “invented” it. Aseptic processing equipment and automation has to be developed from first principles for these applications.
The trend of self-administering injectable drugs and the goal to reduce injection frequency has driven the development of wearable/large volume injectors. These are designed for administering injectable drugs in large volume, typically more than 2 mL, and/or injection over an extended time. Many of these devices use conventional cartridge-based primary drug containers. In connection with the trend towards COC previously mentioned, there are a number of novel devices in development taking advantage of the flexibility of injection mouldings to create patient benefits. Some of these unique features are driving the need for custom automation and custom fill-finish solutions. As the drug substances are denatured by terminal sterilisation, the custom automation and fill-finish need to be accomplished aseptically.
Linked to desire of reduced injection frequency, some daily injections are moving to “depot”-based sustained-release products. The drug is mixed with a polymer, which biodegrades safely to release the drug over many days or weeks. These technologies are typically based around poly lactic-co-glycolic acid (PLGA), which is now used in many US FDA-approved devices owing to its predictable and tuneable biodegradability and biocompatibility (safety profile).3 Such technologies have the ability to deliver small molecules and biologics, including proteins, vaccines, DNA, RNA and peptides. Typically, these devices rely upon some form of reconstitution between a powder (often freeze or spray dried) and a diluent to form a subcutaneous sustained-release gel following injection.
The recent success of covid-19-related vaccines has brought into sharp focus the challenges of cold chain logistics. It is well recorded that cold chain logistics can be challenging, especially in hot and developing nations. Even within developed nations the “last-mile” is a recognised problem leading to cold chain breaches. Drug substances tend to be significantly more shelf-stable at ambient conditions as a powder rather than as a liquid and, clearly, parenteral injections are predominantly liquids delivered through a needle. This has led to the rise in reconstitution devices, which mix a shelf-stable powder with a diluent just prior to administration. These are dominated by lyophilisation within vials or cartridges. Some applications use spray drying as a means of improving the speed of reconstitution (for poorly soluble materials). The reconstitution within vials is a complicated multistep process for caregivers, which can lead to errors. Numerous reconstitution “twin chamber” systems are under development with some form of bypass/valve between the chambers. These enable liquid/liquid or powder/liquid reconstitution just prior to administration. This trend has given rise to the need for precise aseptic powder dispensing. In addition, the physical properties required for solubility tend to go hand in hand with a sensitivity to humidity and poor flow characteristics, meaning these powders tend to be a challenge to dispense from bulk into a primary drug container such as a twin chamber cartridge or novel device.
Another area that has grown in interest is the generation of small diameter extruded depots. In these applications, a small depot similar in size to a grain of rice is first extruded into a continuous length prior to drying and cutting operations. Such devices are clearly limited in the size of the payload. They are typically injected under the skin to deliver sustained-release hormones or vaccines. As above, these drug substances are typically denatured by terminal sterilisation, such that these novel processes must be produced in an aseptic manner.
THE RISE OF CELL AND GENE (ATMP)
One cannot discuss trends within parenteral administration without discussing what tends to be called “cell and gene” in the US and ATMPs in Europe. These therapies encompass a wide range of treatments including genetic editing techniques and chimeric antigen receptor (CAR) T-cell therapies. They use engineered cells to boost a patient’s own immune system to fight diseases such as cancer. These intravenous therapies are currently seeing an explosion in activity with billion-dollar acquisitions, IPOs and investments. It is only a few years (2017) since the FDA made its first approval of a gene therapy.
The growth is very reminiscent of the not-so-distant biologics boom in monoclonal antibodies. By way of example, in 2017 there were reported to be ~2,600 ATMP trials across 38 countries4 and market surveys suggest over 30% annual growth between 2020 and 2027 from around US$2.5 billion (£1.8 billion) to $25 billion (£18 billion).5 In 2020, there were 154 reported trials within the UK alone.6 The technology is moving rapidly from the lab to patients, and that hints at some of the challenges. Poorly defined semi-automatic and manual processes developed by academics in university laboratories are currently being industrialised, scaled and validated. The pharmaceutical sector is a highly regulated environment used to high-volume production, with formal procedures for the simplest of tasks. Knowledge and the guidance are having to be developed for these novel ATMP treatments. Meanwhile the rapid expansion of the sector has led to a skills shortage drawing in staff from traditional high-volume manufacture.
Manufacturing costs and complexity of manufacturing tend to be high in a nascent industry launching products made with an innovative technology. For the ATMP companies, working through the scale-out/scale-up challenge is a priority.
For autologous treatments the batch size is one. Cells are harvested from a patient, modified and expanded and then returned to the patient, typically intravenously. As discussed, laboratory processes can be very manual and there is currently no scale. As such, the introductory price for ATMPs can range from $18,950 (£13,650) to $1.2 million (£869,256) per patient.7 There is a real and present need to reduce the cost of production by a factor of 10–20. The situation is reminiscent of bespoke car production before Henry Ford introduced the production line in 1913.
The jury is currently “out” on what the equivalent efficient production system is for ATMPs. Some believe the future lies in closed single-use ecosystems designed to only run upon dedicated processing machines. This is an Apple business model, whereby all items of the ecosystem need to be procured from a single source. Others are investigating robotic processing within an aseptic isolator, agnostic to the consumable vendors: think open-source Android. What is clear, is the future will require significant amounts of automation technology. The goal is to create a repeatable manufacturing platform for ATMPs that many companies can replicate. Aseptic equipment manufacturers have their part to play in responding to this new market need with innovative solutions.
A SUMMARY OF ASEPTIC AUTOMATION TRENDS
The parenteral market for aseptic automation is changing, as shown pictorially in Figure 3; this is being driven by a number of factors. As described, this is partly due to the trend of new medicaments being higher value but lower volume. Tubs are now available from numerous vendors to hold arrays of primary drug containers. The introduction of COC primary drug containers and large-volume (on-body) devices is requiring custom automation solutions. Depot-based and reconstitution-based technologies also require custom automation solutions, and often, this also includes the need for aseptic powder dispensing. The current explosion of ATMP application is also driving the need for automation innovation – this is particularly the case for autologous applications where the batch size is one. Overlaid on top of these device-driven trends is the need to minimise the number of manual operations to reduce particulates and product-to-product variability.
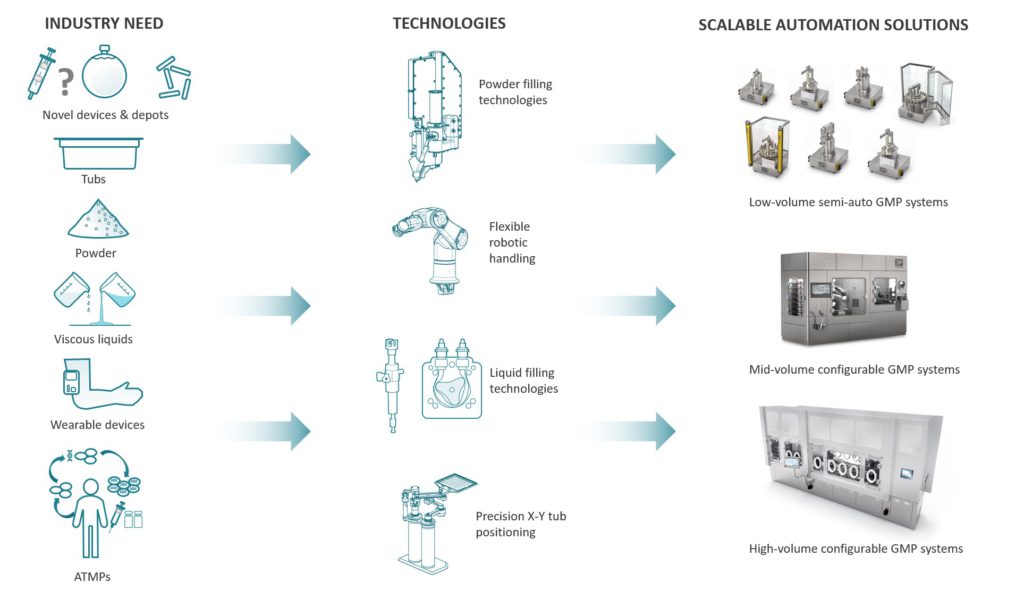
Figure 3: Drivers for change in aseptic automation and 3P’s response.
“By creating a configurable platform comprising
state-of-the-art technologies, these systems address the need to provide solutions for new products/devices, such as an aseptic spray-dried powder into a dual chamber syringe, in a way not yet seen by the industry.”
ONE COMPANY’S RESPONSE
As a custom automation house, 3P is, and has been, well placed to respond to the above trends and challenges. A significant proportion of the company’s work remains the development of highly engineered custom solutions for its clients. These systems are developed to enable clients to scale-out and/or scale-up, depending upon need. The automation is developed specifically for the needs of the client’s product. Some recent custom examples include:
- Aseptic ram-die and aseptic twin screw extrusion of depots
- Aseptic cutting of depots
- Manufacture of COC-based primary drug containers (for terminal sterilisation)
- Helium leak testing of primary drug containers
- Aseptic liquid and powder filling of standard and custom primary drug containers: – Including device assembly
- Aseptic cell processing
- Vision inspection systems of the product in Grade A.
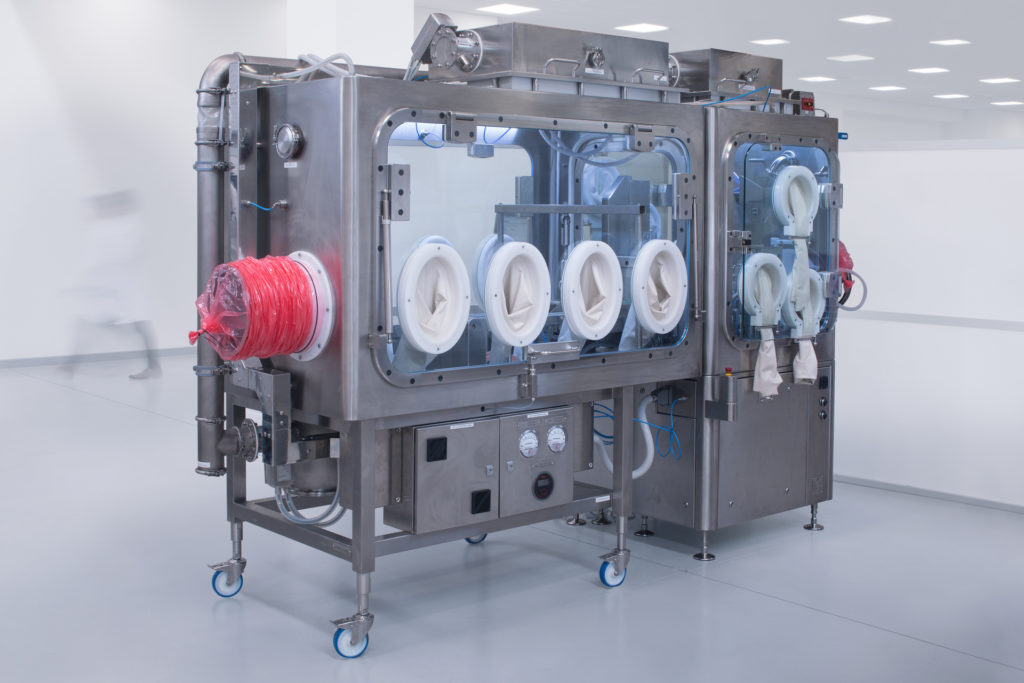
Figure 4: Mid-scale robotic fill-finish (3P’s custom R1000).
Like many automation houses, 3P has a range of “standard” aseptic modules that can be employed as required, each an evolution in known technologies with a focus on low-particulate generation and class-leading precision. Hence, the company has a range of modules to de-lid tubs, to manipulate tubs, to de-nest and to re-nest devices into tubs. 3P has a range of pumps and lift/rotate modules to enable aseptic liquid dispensing (and some unique powder dispensing technology – see Figure 4). There are stopper feed bowls and stoppering modules (including vacuum). Finally, the company has low-particulate generating crimping modules. Systems can then be “glued together” by its robotic solutions and placed into an isolator from a range of isolator vendors with a range of sterilisation methods (iHP/vHP/HPV etc). To this end, 3P has focused on aseptic robotic solutions from a particular vendor; the company’s default is to use the Stäubli TX2 range of aseptic six-axis collaborative robots, so called “cobots”. The cobot feature enables the safe integration with manual operations and enables the guard line to be within the reach of the robot (to minimise the size of any isolator). These are also sealed against hydrogen peroxide sanitisation and have an exterior that is a specialist non-shedding white coating. For some space-constrained tub-based solutions, 3P has developed its own compact “crabot” robot. This system has a small footprint and can remove devices from an ISO-tub filled with devices.
3P’s award-winning Fill2Weight powder dispensing technology (originally developed for inhaled applications), has been used in aseptic processing applications for over 10 years. The narrow form factor is ideal to maintain unidirectional air flow within an isolator and it is fully sealed for sanitisation or clean in place (CIP). Expertise has been developed to ensure powder is not released, even within low-humidity environments and under unidirectional air flow. 3P’s patented aseptic gravimetric powder dispensing is one of the company’s unique offerings to the market.
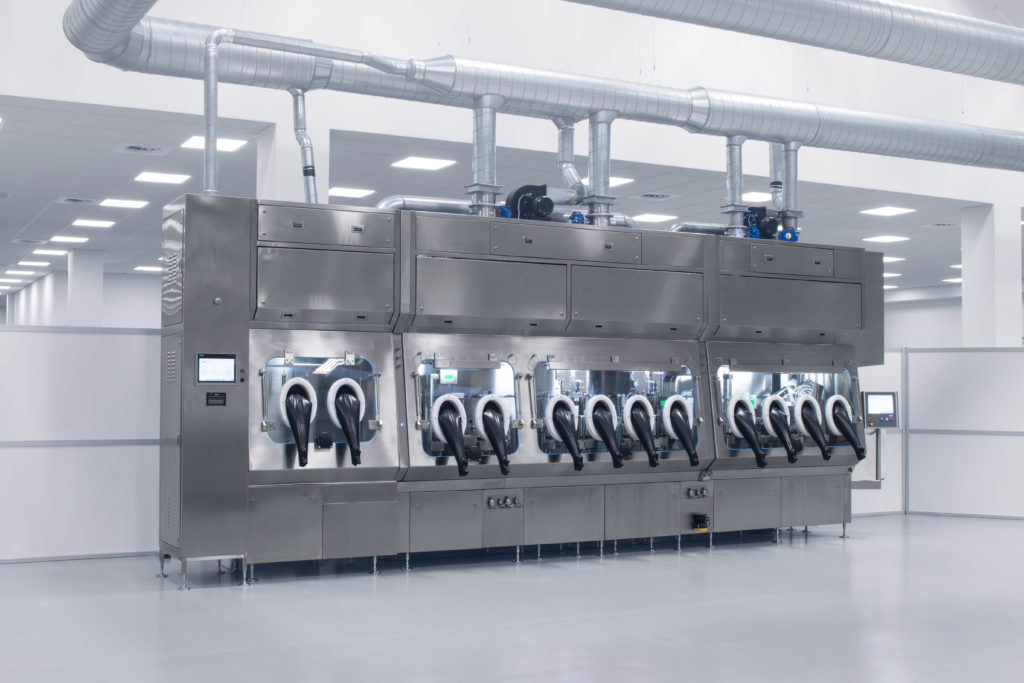
Figure 5: Commercial-scale robotic fill-finish.
With the need for flexible tub-based fill-finish systems, 3P repackaged some of its “standard” fill-finish modules described above, into a flexible multiformat robotic powder or liquid-filling system with integrated stoppering/capping and crimping – the F2/F5 range of fill finish equipment (Figure 5). By creating a configurable platform comprising state of- the-art technologies, these systems address the aforementioned need to provide solutions for new products/devices, such as an aseptic spray-dried powder into a dual chamber syringe, in a way not yet seen by the industry. This filling platform is then encapsulated within an isolator with tub loading via NTT (Neweco, Warsaw, Poland) or a decontamination chamber to suit the product and operator protection requirements. While these could be gloveless systems, clients still prefer the insurance that gloves provide, in case a rare intervention is required.
To address the very low-volume ATMP requirements, the “standard” modules described previously have been repackaged as stand-alone pieces of semi-automatic machinery. These can be single stations or integrated with a small indexing carousel to increase throughput. They are designed to be very compact for integration into small isolators and to be operated via glove ports.
CONCLUSION AND OUTLOOK
It is clear that it is an exciting time for the aseptic automation sector. Automation vendors need to embrace the changes brought about by a wide range of factors:
- New and innovative injectable devices taking advantage of the trend for home administration and caregiver convenience.
- A move from glass to plastic (COC) primary drug containers. This is partially led by robustness against cryogenic temperatures, advances in polymers, market acceptance and also because they enable additional features to be moulded onto the primary drug container.
- A reduction in batch sizes taken advantage of by tub-based supply of primary drug containers. This, in turn, leads to a requirement for more compact fill-finish lines to reduce the size of very costly aseptic clean room facilities.
- A reduction in human variability (and error) by automating manual processes. The drive to robotics and automation is also driven by a desire to reduce operators and gloves to reduce particulate generation.
- A desire to avoid cold chain is leading to investment in engineered particles such as those produced by spray drying, bulk freeze drying and cryo-milling. This, in turn, is leading to a growth in precision aseptic powder-filling technology.
- Huge investments are being made in cell and gene therapies (ATMPs), which have only recently emerged from the lab. The commercialisation of these ultra-low volume and ultra-high value products is being hampered by the lack of “opensource” (think the Apple ecosystem) automation solutions.
All the above has led to an explosion of innovative aseptic automation solutions, of which 3P innovation is at the forefront with a wide range of liquid and powder fill-finish automation platform technologies. These platform technologies can be configured for benchtop solutions for clinical and ATMP applications. Similarly, they can be integrated within a higher volume robotic solution, typically with a tub-based infeed. Aseptic liquid and powder dispensing can be added to these fill-finish lines as required. Finally, custom modules can be developed as required to deal with innovative injectable devices.
What is clear is the outlook for aseptic automation companies is one of innovation and change. Fun times ahead!
REFERENCES
- Sandle T, “People in Cleanrooms: Understanding and Monitoring the Personnel Factor”. Journal of GxP Compliance, 2014, Vol 18, pp 1–5.
- Keller M et al, “Optimized Robot Systems for Future Aseptic Personalized Mass Production”. Procedia CIRP, 2018, Vol 72, pp 303–309.
- Makadia HK, Siegel SJ, “Poly Lactic-co-Glycolic Acid (PLGA) as Biodegradable Controlled Drug Delivery Carrier”. Polymers (Basel), 2011, Vol 3(3), pp 1377–1397.
- Ginn SL et al, “Gene therapy clinical trials worldwide to 2017: An update”. The J Gene Med, 2018, Vol 20:e3015.
- “Global Cell and Gene Therapy Market”. Research Report, BIS Research, 2021.
- “Cell and Gene Therapy Catapult ATMP Clinical Trials Database 2020”. Company Web Page, Cell and Gene Therapy Catapult (Accessed April 26, 2021).
- Seoane‐Vazquez E, Shukla V, Rodriguez‐Monguio R, “Innovation and competition in advanced therapy medicinal products”. EMBO Mol Med, 2019, Vol 11(3):e9992.