To Issue 150
Citation: Knappett B, “High-Throughput Screening to Accelerate Nanomedicine Discovery.” ONdrugDelivery, Issue 150 (Jul 2023), pp 12–15.
Ben Knappett discusses how the successful combination of microfluidics with automation can provide rapid and cost-effective high-throughput lipid nanoparticle technology formulation screening, and how this approach could play a significant role in advancing the development of new nanotherapies.
“The field of nanomedicine holds promise for overcoming the undesirable properties of many conventional drugs that limit their clinical use, including poor pharmacokinetics, restricted bioavailability and high toxicity.”
The widespread introduction of covid-19 mRNA vaccines was underpinned by lipid nanoparticle (LNP) technology, and the success of this rapid roll-out has solidified the viability of nanomedicines, attracting new funding and interest in this area. The market continues to boom, and recent scientific breakthroughs have proven LNPs to be highly effective drug delivery systems for a range of therapy types. The ability to screen large numbers of different formulations is essential for optimising numerous performance characteristics that depend on a specific particle size, shape and structure. Automated systems are ideal for this screening, surmounting the challenges associated with typical low-throughput LNP preparation methods.1
EXPLORING THE POTENTIAL OF NANOMEDICINES
The field of nanomedicine holds promise for overcoming the undesirable properties of many conventional drugs that limit their clinical use, including poor pharmacokinetics, restricted bioavailability and high toxicity.2 Using LNPs as drug delivery systems is a revolutionary way of improving the therapeutic index of a drug, and the viability of this technology has recently been validated by the successful development of LNP-mRNA covid-19 vaccines. As a result, this approach has received significant research interest and offers key advantages over other drug delivery options, enabling the encapsulation of a range of payloads with high efficiency, stabilising the API and helping to deliver it to target cells (Figure 1).
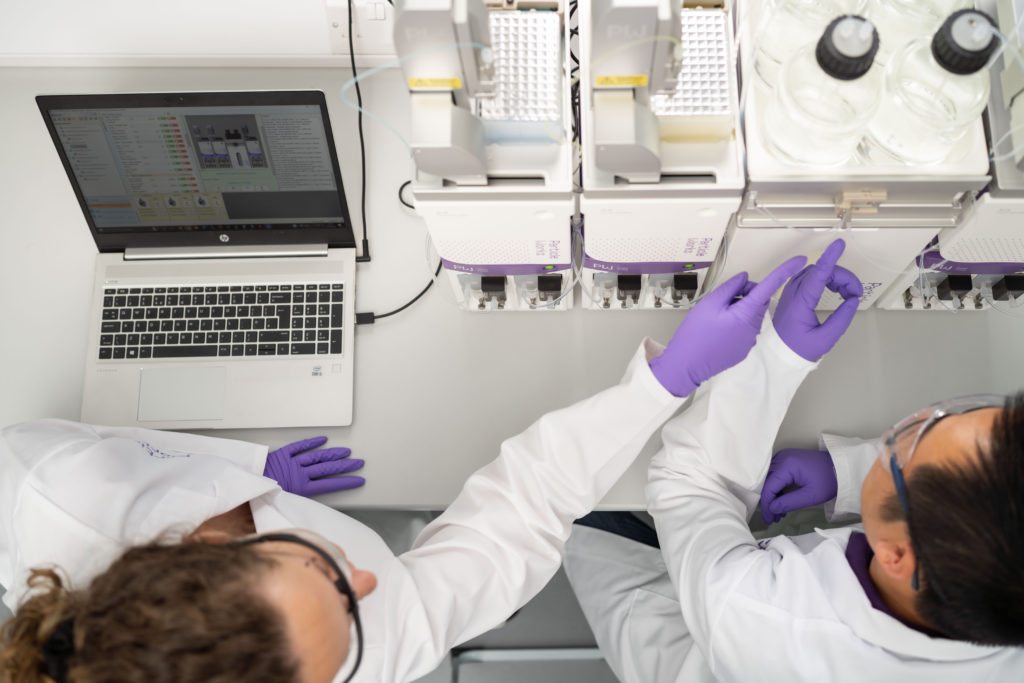
Figure 1: Novel systems combining microfluidics with automation provide high-throughput LNP formulation screening to accelerate the creation of pioneering genetic medicines and cancer treatments.
“There is a clear demand for a platform that can rapidly synthesise trial nanoformulations in a controlled manner, allowing efficient screening and process parameter adjustments.”
The success of LNP medicines is widely acknowledged to lie in the specific formulation of the particle, as this can significantly affect the therapeutic efficacy. The optimal formulation for each application is vital to turn promising biologics into effective and viable therapeutics – whether for a vaccine, gene therapy or cancer treatment – and reproducibly creating uniform particles with finely tuned performance characteristics is vital to achieving this.3 Furthermore, nanomedicines are intended for clinical applications, meaning that LNPs must be consistent and safe for use in humans.
FINDING THE PERFECT BALANCE
LNPs must be carefully engineered to ensure that they can perform the complicated series of actions necessary for effective drug delivery – including transport across cell membranes and intracellular release – while keeping their therapeutic cargo intact. Several different factors may affect the success of an LNP, and must be controlled by altering the liposomal formulation of the particles to achieve the ideal performance criteria. Particle size affects both uptake and delivery – with the best performing formulations being between 75 and 95 nm in diameter4,5 – and the polydispersity index, a measurement of the uniformity of particle sizes, must have a value of 0.2 or below to be deemed acceptable.6
The biological performance of an LNP largely depends on four lipidic components: ionisable lipids, phospholipids, PEGylated lipids and cholesterol.1,7 Both the molar ratio and type of lipid material can be altered, with multiple options currently in clinical use (Table 1).
Lipid type | Options in Clinical Use |
Ionisable | SM-102 MC3 9A1P9 |
Phospholipid | Phospholipon-90G DSPC |
PEGylated | DMG-PEG2000 DOPE-PEG2000 DSPE-PEG2000 DSPE-PEG5000 |
Cholesterol | – |
Table 1: Lipid categories.
Many different LNP factors can be fine-tuned, such as cargo concentration, length or sequence, as well as the charge ratio of the particle itself. Process parameters – including total flow rates, reagent mixing kinetics, pH and type/concentration of buffer – can also be adjusted. To identify the “sweet spot” of particle performance, small quantities of every permutation of formulation parameters must be synthesised. Physiochemical characterisation of each batch is then screened, before the most promising nanoformulations are selected to take forward. This cumbersome process is largely reliant on trial and error, and requires potentially billions of investigations. The experimental process must also be consistent and validated, quickly racking up high materials and consumables costs, as well as incurring heavy demands on labour and time.
“LNP medicines have the potential to transform patient care in the near future.”
AUTOMATION FOR IMPROVED THROUGHPUT AND CONTROL
Low-throughput, manual screening introduces large workflow bottlenecks, delaying downstream assays and characterisation steps. In the absence of automation in both upstream and downstream stages, there is a danger of missing the perfect nanoformulation, hindering discovery.3 There is therefore a clear demand for a platform that can rapidly synthesise trial nanoformulations in a controlled manner, allowing efficient screening and process parameter adjustments.
Microfluidic devices can achieve this by manipulating fluids on the microlitre scale, generating reproducible and monodisperse nanoformulations possessing tightly controlled physical properties. However, most microfluidic systems used for formulation screening only allow one experiment to be performed at a time before experimental parameters need to be changed manually. As a result, this method had not been able to offer sufficient automation until recently.8
The latest automated microfluidic platforms overcome this to enable effective and efficient high-throughput generation of numerous LNP formulations. These innovative platforms significantly accelerate screening timeframes, offering superior process consistency, increased automation and minimised running costs (Figure 2). They are compatible with 96-well plate formats – completing up to 96 experiments in as little as six hours – to seamlessly fit with existing upstream and downstream workflows, permitting easy sample transfer between all stages of particle production.
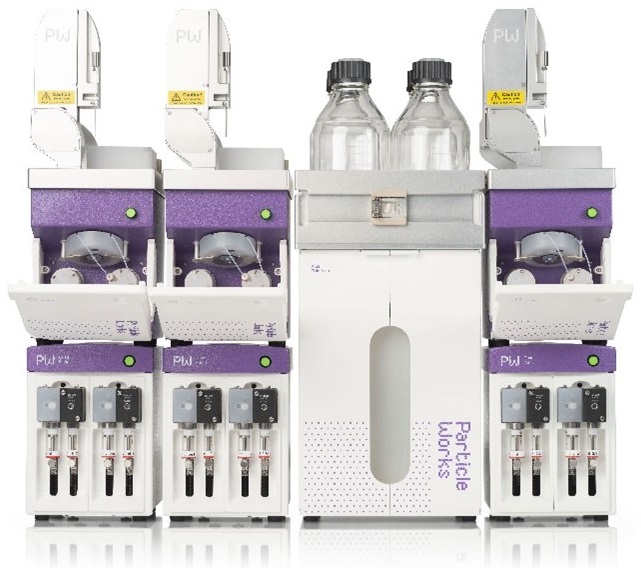
Figure 2: Example of an automated microfluidics-based high-throughput formulation screening platform.
These platforms feature reusable glass microfluidic chips and automatic washing between experiments to ensure that there is no cross-contamination, which enables complete system recovery and highly reproducible LNP formulation following the addition of unstable samples (Figure 3). They therefore offer high levels of flexibility with minimal manual intervention by the user. A single system can be used for both process optimisation and continuous production, simplifying the transfer from early-stage particle screening to scaled-up production and empowering researchers to accelerate nanomedicine discovery.
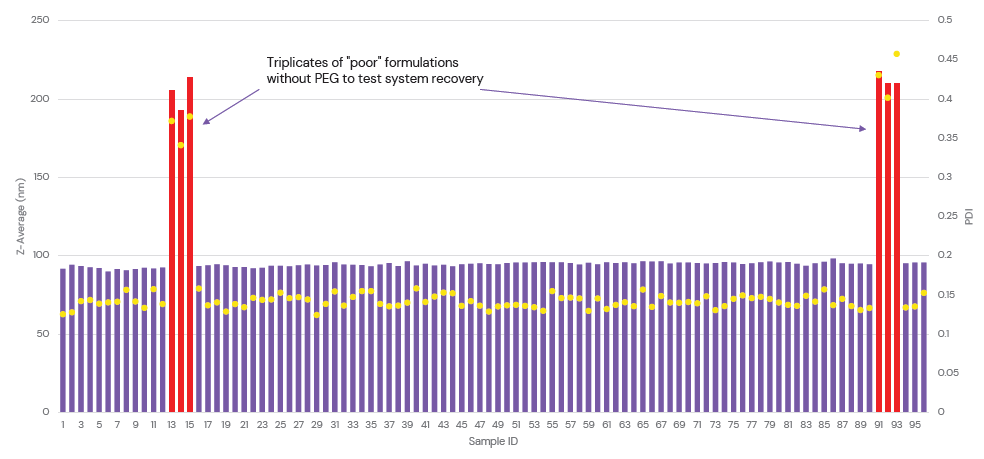
Figure 3: System recovery of the ALiS automated microfluidic platform was tested by the addition of six spike sample formulations with no PEGylated lipid. There was a null effect on the reproducibility of subsequent samples, demonstrating the efficacy of the automated wash between experiments.
A HOPEFUL FUTURE FOR NANOTHERAPIES
LNP medicines have the potential to transform patient care in the near future, and encapsulating biological material is now an integral part of the early-stage development of genetic medicines and vaccines. To bring an LNP-based therapeutic to the global market, researchers need to consider how quickly they can go from screening nanoformulations to the clinic and, ultimately, on to commercialisation. To synthesise LNPs reliably and reproducibly with optimal performance characteristics, there is a clear requirement for automated high-throughput platforms that offer excellent synthesis control. Novel microfluidics systems meet this need, combining controlled synthesis of LNP formulations with automated high-throughput formulation screening to minimise costs and shorten development timelines. These innovative platforms are already helping to accelerate the development of therapeutics that rely on lipid-based drug delivery mechanisms, advancing the creation of genetic medicines and cancer treatments to help address some of the world’s urgent healthcare challenges.
REFERENCES
- Cui L et al, “Development of a high-throughput platform for screening lipid nanoparticles for mRNA delivery”. Nanoscale, 2022, Vol 14, pp 1480–1491.
- Beltrán-Gracia E et al, “Nanomedicine review: Clinical developments in liposomal applications”. Cancer Nano, 2019, Vol 10, p 11.
- Zaslavsky J, Bannigan P, Allen C, “Re-envisioning the design of nanomedicines: Harnessing automation and artificial intelligence”. Expert Opin Drug Deliv, 2023, Vol 20(2), pp 241–257.
- Tenchov R et al, “Lipid Nanoparticles from Liposomes to mRNA Vaccine Delivery, a Landscape of Research Diversity and Advancement”. ACS Nano, 2021, Vol 15, pp 16982–17015.
- Hassett KJ et al, “Optimization of Lipid Nanoparticles for Intramuscular Administration of mRNA Vaccines”. Mol Ther Nucleic Acids, 2019, Vol 15, pp 1–11.
- Danaei M et al, “Impact of Particle Size and Polydispersity Index on the Clinical Applications of Lipidic Nanocarrier Systems”. Pharmaceutics, 2018, Vol 10(2), p 57.
- Hald Albertsen C et al, “The role of lipid components in lipid nanoparticles for vaccines and gene therapy”. Adv Drug Deliv Rev, 2022, Vol 188, p 114416.
- Zhao X et al, “Microfluidic Generation of Nanomaterials for Biomedical Applications”. Small, 2020, Vol 16(9), p 1901943.