Citation: O’Prey C, “Sustainability, Waste & Remanufacturing in the Medical Sector”. ONdrugDelivery, Issue 126 (Oct/Nov 2021), pp 12–16.
Cormac O’Prey discusses some of the sustainability challenges facing the medical sector, such as how to make re-use and remanufacturing viable for high-volume, low-value products, and how answers can be found by looking to successful solutions deployed by other industries.
Healthcare contributes 4–5% of all global greenhouse gas emissions, with inhalers comprising a significant portion of that.”
INTRODUCTION
“Sustainability”, “recycling” and “re-use” are not new ideas. Many people can still remember returning lemonade bottles for 10p apiece and putting out empty milk bottles for the milkman to collect. Some still do. So what happened? When did “use and return” become “chuck and forget”? More importantly – how do we change back? And why should we? Can we combine forgotten 20th century wisdom with 21st century circular economy innovation to stem the tide of rubbish that is clogging rivers and seas around the world? And what about us in the medical industry, and specifically drug delivery? What can we do to respond to growing public, government, patient and healthcare provider demands that we clean up our act? And how can we do it while controlling risk, without compromising safety standards and, crucially, while maintaining a profitable business?
With COP26 taking place as this article goes to press, there has never been more pressure on industry to reduce its environmental impact. Historically, with the priority on minimising patient risk, the healthcare sector has been considered exempt from sustainability demands. However, there is strong evidence now that healthcare is, in fact, a major contributor to global pollution and rising CO2 levels. If ranked alongside countries, the healthcare industry would be be the fifth-largest emitter on the planet,1 so that supposed exemption no longer holds true.
With the implementation of net zero CO2 targets set for 2030,2 major healthcare providers and suppliers are now taking sustainability very seriously. As UK NHS Chief Executive Sir Simon Stevens said, “While the NHS is already a world leader in sustainability, as the biggest employer in this country, comprising nearly a tenth of the UK economy, we’re both part of the problem and part of the solution.”
Achieving “sustainability” in the medical sector generally covers reducing plastic product and packaging waste, CO2 and CO2 equivalent (CO2E) emissions, and energy and water usage. Healthcare contributes 4–5% of all global greenhouse gas emissions, with inhalers comprising a significant portion of that – inhalers account for 3–3.5% of the NHS’s carbon footprint3 and 28% of GSK’s CO2E emissions (amounting to 8.4 MT) come from pressurised metered dose inhaler (pMDI) cannister propellants alone. As such, prioritising alternative, lower global warming potential (GWP) propellants makes sense, and progress towards this goal is being made. By volume, the GWP of current propellants is equivalent to over 3,000 units of CO2, whereas some proposed alternatives are equivalent to less than one unit.
Changing the industry’s preferred inhaler propellant will not affect product and packaging waste, however. Device manufacturers can also reduce their environmental impact by adopting sustainable design and manufacture. According to the United Nations, an 80% reduction in CO2E emissions is achievable by adopting sustainable manufacture.4 Although waste from devices and packaging is a lesser contributor to CO2E emissions, they still represent a significant problem and this is the challenge we will focus on in this article.
“Device design is intimately linked with sustainable manufacturing and reprocessing, with the product design requirements needing to reflect how a product will be managed at the end of its life.”
SUSTAINABLE DESIGN AND MANUFACTURE
What is “sustainability” and how do device manufacturers achieve it? With the massive growth in interest in sustainability, variations in terminology have arisen, leading to misunderstandings and confusion. For example, “remanufacture” of single-use devices (SUDs) means very different things according to US FDA Guidance for Industry5 and the EU Medical Device Regulation (MDR).6
According to the World Commission on Sustainability, “Sustainable development seeks to meet the needs and aspirations of the present without compromising those of the future.” Sustainability in the context of drug delivery devices can be broadly divided into two disciplines – sustainable product design and sustainable manufacture – where CO2E and environmentally damaging material waste is minimised. Device design is intimately linked with sustainable manufacturing and reprocessing, with the product design requirements needing to reflect how a product will be managed at the end of its life. To achieve a stable sustainable device manufacturing strategy in the long term, these elements must work in harmony, ideally offering a solid business incentive to manufacturers. Looking at successful examples of sustainable design and manufacture readily shows that a sustainable business case is just as important as a sustainable device design for long-term viability.
To develop and disseminate sustainable strategies, and to try and add some consistency and consensus, the British Standards Institution (BSI) and the International Standards Organisation (ISO) have led the way on how sustainability can be generally implemented in product design and manufacture. The BS8887 series of standards, including “BS/ISO 8887-2: Design for Manufacture, Assembly, Disassembly and End-of-Life Processing (MADE) Terms and Definitions”,7 suggests a framework and a set of standard terms to describe the relevant processes.
The framework suggests a range of alternative – but not mutually exclusive – routes for products and components that have reached the end of their useful lives based on their “residual values”. At the top, products that are suitable for remanufacture represent the ideal for sustainability – a product that can be recovered with the maximum residual value intact and returned to the market in as-good-as-new condition at the original retail cost with warranties and at minimum cost to the manufacturer. For example, manufacturers of medical imaging equipment regularly have a significant portion of the component inventory used in new machines coming from previously used versions – with corresponding savings on manufacturing cost and waste generation. Going down through the options in the framework represents progressively lower levels of recovered value and therefore lower economic incentives for manufacturers to commit to these strategies.
BACKGROUND AND DRIVERS FOR CHANGE
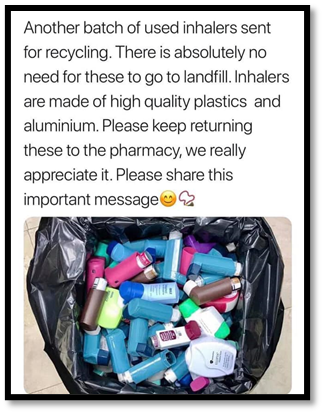
Figure 1: A 2019 Facebook post shared 20,000 times.
So, if most of the environmental damage from inhalers comes from propellant gasses, why are we concerned with waste from used devices? The simple answer is because, as with many other products in many other industries, throwing away large quantities of used medical products has become unacceptable. Inhaler use in the UK alone is set to double from 2006 levels of 35 million to 70 million by 2030. With this increase in inhaler use, governments, pressured by public opinion and environmental groups, are forcing manufacturers to reduce their environmental impact. This is increasingly leading to restrictions being placed on waste, with manufacturers being held accountable for their used products.
In the medical sector, clinicians are now considering the environmental impact of therapies in their prescribing decisions, and the NHS is making environmental performance a key requirement of suppliers, targeting a 50% reduction compared with 1990’s waste levels by 2028. Furthermore, instances of patients showing reluctance towards using their inhalers because of the environmental impact have been reported, and social media campaigns have been started to force medical product manufacturers to take care of their used products (Figure 1).
On a more positive note, medical device requirements documented by the MDR, FDA and UK Medicines and Healthcare products Regulatory Agency now consider how devices can be re-used without compromising safety, and medical device regulators have been directed to help manufacturers find more sustainable solutions. The language has shifted from patient safety as the sole consideration to the need for manufacturers to balance such with environmental impact. In recent industry conferences, such as Respiratory Drug Delivery and Drug Delivery to the Lungs, the topic of sustainability featured heavily, and collaborative working groups, such as the End-to-End Sustainable pMDI Forum sponsored by Aptar (IL, US) and Pharmaserve North West (Runcorn, UK), are being set up to find solutions.
The good news for medical product manufacturers is that many comparable solutions have already been established in other industries. Circular design and manufacture has been in use in the medical sector for decades – although more for financial benefit rather than environmental reasons. Indeed, the financial case for circular manufacturing may become more broadly relevant in the near future as the EU is due to publish the documentation for its green finance taxonomy in 2022, which aims to provide a framework for companies and public authorities to use “green bonds” to raise capital for large-scale sustainability investments.
High-value, low-volume medical imaging equipment remanufacturing has successfully recovered and re-used 80–90% of the components from previously used machines, including the “heavy iron” components such as magnets, motors and structural components that constitute much of the residual value. Indeed, anticipating re-use over many cycles, General Electric (MA, US) now specify these components with up to a 40-year design life. We can learn from this.
Manufacturers of low-value, high-volume medical products, such as surgical instruments, have tried to implement circular manufacturing, but have seen more mixed success, in some cases struggling to compete with third-party remanufacturers who can recover, clean, test and resell used devices at a lower cost than original equipment manufacturers (OEMs).8 Partnerships between OEMs and third-party remanufacturers have been set up to play to the strengths of each partner, which is how some believe the industry will evolve. Outside the medical sector, manufacturers such as Nokia have pioneered designs that are optimised for multiple lifetimes, with parts likely to become worn or damaged being designed to be cheap and easy to replace. Another example is the chair manufacturer Orange box, which designs its core components to be durable enough to last multiple lifetimes, with covers designed to be removed and replaced quickly using zip fasteners.
On the challenging aspect of how to manage “reverse logistics”, where products are recovered and returned to the OEM for reprocessing, innovators in the medical sector have struggled with low device return rates, low yields of usable product, unpredictable recovery rates and components in poor condition. Reverse vending machine makers Tomra (Asker Municipality, Norway) have automated the process of recovering plastic drinks bottles and soda cans to reduce costs and have incentivised customers to return such waste.9 Even recycling these low-value products now makes good business sense, and could be looked into as part of a potential solution in the medical sector.
WHAT DOES GOOD SUSTAINABLE MANUFACTURING PRACTICE LOOK LIKE?
Some drug delivery device manufacturers have operated recovery and recycling schemes in which inhalers are returned to pharmacies and separated into plastic and metal components. The aluminium in pMDI cans is recovered and plastic inhaler bodies are recycled as, amongst other things, benches and playground equipment. While such schemes reduced the number of devices going to landfill, Figure 2 shows how this sort of recycling offers little opportunity to recover much residual value and little in the way of financial return. With only a 0.3% return rate, GSK replaced its scheme with a more comprehensive approach in 2020.10
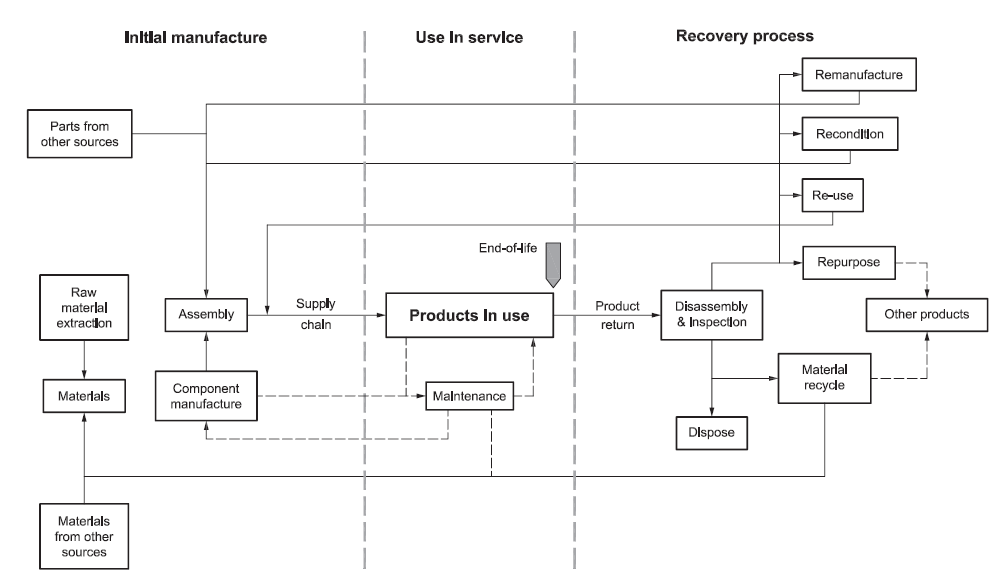
Figure 2: Cascade diagram shows decreasing residual value of used components from BS 8887-2:2009 standard (reproduced with kind permission from BSI).
“Designing smart devices for multiple lifetimes and considering multiple remanufacturing cycles during the design phase could address the issue of affordability for healthcare providers.”
This leads into the industry’s present conundrum – the question of how to combine a sustainable device design that supports value recovery and minimises waste and CO2 emissions with a practical and effective recovery and re-use strategy. We know what we want to do, but we haven’t quite figured out how to do it.
Progress in device design is being made with DuPont (DE, US) researching materials suitable for extended lifetimes and H&T Presspart (Blackburn, UK) launching a new plasma coating process for pMDI cannisters to extend their lifespan (discussed in more detail by H&T Presspart, here). The introduction of smart devices with their higher manufactured cost and their greater potential for environmental damage, due to their embedded electronics and batteries, is an interesting case and could stimulate the adoption of design for remanufacture and circular manufacturing models.
Designing smart devices for multiple lifetimes and considering multiple remanufacturing cycles during the design phase could address the issue of affordability for healthcare providers. If a device is too expensive to manufacture for a single three-month use cycle, amortising the cost over several lifetimes could reduce total cost of ownership to acceptable levels. Furthermore, if you can recover and remanufacture such a device efficiently, you have an opportunity to assess its condition and ensure that its performance over its next lifecycle will be satisfactory. By doing so, you can manage patient risk and get to sell the device again without the cost of having to make another one. There is also an opportunity to analyse a used device for issues, performing a valuable post-market surveillance function, and accessing embedded smart data could give valuable insights into patient population behaviour.
Clearly, when designing a device for multiple lifetimes, issues of robustness and degradation that do not apply to SUDs need to be considered. However, doing so does afford the opportunity to invest more in the design while still providing it at an affordable cost. Paradoxically, to reduce plastic waste you may need to make the design more robust by adding more plastic to its constituent components so that they last longer.
Examples of successful sustainable designs can be found in other industries, and the characteristics that make them work can be analysed and applied to medical devices. In device design, sustainability must be included in the requirements specification from day one in order to ensure that it drives the design development. Sustainability cannot be applied as an afterthought. Identifying the “core” of a device – the elements of the design that can be economically recovered with sufficient residual value and in good condition – is a key step.
Counterintuitively, existing designs may need to be split to separate the parts that can be re-used from those that cannot. For example, designing in sacrificial covers that protect valuable mechanisms but cannot be re-used themselves may make sense. Reasons for rejecting used components for remanufacture can include damage, wear, contamination, discoloration and even fashion. This may go against established design for manufacture and assembly (DFMA) principles and lead to an increase in part count but will reduce waste volumes overall, and of course, there are options, including biodegradable materials such as those supplied by Celanese (TX, US), to ensure that rejected parts do not contribute to plastic waste.
Adopting a modular design approach can be helpful in this respect, and can also provide some flexibility in updating design elements that have become obsolete. In this case, a “spiral” manufacturing model may be a better fit than a circular one, as market expectations are constantly changing such that remaking what was made yesterday may not be an option. Long-term stable designs are those where manufacturers can be confident that old parts will still be useful in the future. The Ellen MacArthur Foundation’s (Cowes, UK) “Upstream Innovation – A Guide to Packaging Solutions” contains useful guidance on packaging design that could be useful in the medical sector.
Effective recovery and remanufacture is the other side of the equation and, just as in DFMA, needs to work in concert with the product design. So far, remanufacturing has largely been restricted to high-value, low-volume products because the process is largely slow and labour intensive. This is acceptable for multi-million dollar computed tomography (CT) scanners, but is unsuitable for mass-produced disposable drug delivery devices, such as inhalers or autoinjectors. To address this, research groups in Beijing (China) are investigating autonomous remanufacture to increase throughput and reduce costs, and the UK recycling industry now has advanced, high-speed waste recognition and sorting robots that are capable of recognising, picking, orientating and positioning up to 40 different types of device. Naturally, the design of devices must consider this, developing devices that can be dismantled without damage, maximising the recoverable yield, but that still prevent patients from accidentally taking their devices apart.
“A “spiral” manufacturing model may be a better fit than a circular one, as market expectations are constantly changing such that remaking what was made yesterday may not be an option.”
Medical device recycling schemes must also contend with historically low return rates. To tackle this, some companies have introduced reverse vending machines, such as those made by the aforementioned Tomra, which recognise returned products, inspect them, categorise them and reward users for returning them. Identifying individual devices is possible, helping to address the issue of traceability and reporting on what has been recovered before it goes further into the recovery process. Successful remanufacturing tends to feature a closed loop with customers so that rates of return and the age and condition of returned devices can be managed. This suggests that one-in-one-out strategies for devices, where replacements for prescription devices are issued on return of previously used ones, are ideal, but we must be realistic about likely return rates – patients lose, forget and damage devices and cannot be denied their medicine if they fail to return an old device. With environmental concern increasing among the public, participation in waste reduction schemes could be high if designed so that incentives are added and barriers to participation are removed.
Once recovered, remanufacturers need to determine how to re-use devices to meet regulatory demands for risk management and traceability while maximising yield and ensuring hygiene. There are established cleaning regimes, such as “AAMI TIR30:2011 (R2016) – A Compendium of Processes, Materials, Test Methods, and Acceptance Criteria for Cleaning Re-usable Medical Devices”, that can help.
FUTURE DEVELOPMENTS
There can be no doubt that there is still a lot of work to do in introducing sustainable design and manufacture in the medical sector, but neither can there be any doubt that it must be done. Manufacturers are understandably wary of changes to their operations that are as fundamentally disruptive as introducing sustainable manufacturing, and our industry is characteristically risk averse for very good reasons. Therefore, the question is how to introduce change at an acceptable rate and risk level, as well as doing so in such a way that the benefits can be demonstrated in limited trials to the entire organisation before fully committing to wholesale change.
Again, other industries can help by showing the way. Lifecycle analysis techniques can create a baseline against which change can be measured and improvements evaluated. This provides compelling, objective evidence of improvement, and successful trials in domestic kitchen products have shown a full return on investment after introducing remanufacturing inside one year. There are many organisations set up to provide support, including the Association of Medical Device Remanufacturers, the European Centre for Remanufacture, the Ellen MacArthur Foundation (who include AptarGroup among their members), the BSI, the ISO, the Nordic Centre for Sustainable Healthcare and the International Pharmaceutical Aerosol Consortium.
There is a developing international consensus, including collaboration between the ISO, the BSI and the Chinese national standards bodies. Furthermore, automated remanufacture of high-volume, low-value products, essential for reaching sustainability in medical products, is under development with UK Universities and research groups alongside groups in China – the 5th International Workshop on Autonomous Remanufacturing was held on October 16–17, 2021 at Beihang University, Beijing.
REFERENCES
- “Greener NHS Campaign to Tackle Climate ‘Health Emergency’”. UK NHS, Jan 2020.
- “Protecting and restoring the planet’s health to protect and improve people’s health”. GSK website, accessed Oct 2021.
- Tennison I et al, “Health Care’s Response to Climate Change: A Carbon Footprint Assessment of the NHS In England”. Lancet Planet Health, Feb 2021, Vol 5(2), pp E84–E92.
- Nasr NZ, Russel JD, “Re-defining Value – The Manufacturing Revolution”. UN International Resource Panel, Nov 2018.
- “Draft Guidance for Industry: Remanufacturing of Medical Devices”. US FDA, Jun 2021.
- “MDR – Article 17 – Single-use Devices and Their Reprocessing”. EU MDR, Jul 2019.
- “Design for Manufacture, Assembly, Disassembly and End-Of-Life Processing (MADE) – Terms and Definitions”. BSI, Apr 2009.
- “Refurbished Medical Equipment Market – Global Industry Analysis, Size, Growth, Share, Trends, Segmentation, Opportunity and Forecast 2020 to 2025”. MedGadget, Jul 2020.
- “Tesco in Slovakia Selects TOMRA as Reverse Vending Supplier for Upcoming Deposit Return System”. Tomra, Aug 2021.
- Clews G, “Inhaler Recycling Scheme that Cut Carbon Emissions Equivalent to More Than 8,500 Cars is Scrapped”. Pharm J, Jul 2020.