To Issue 133
Citation: Welch W, “Advancements and Evolution of Power Sources in Drug Delivery”. ONdrugDelivery, Issue 133 (May 2022), pp 57–60.
William Welch considers the evolution of compressed gas alternatives to spring power for single-use drug delivery devices.
The single-use autoinjector as we know it today, beginning with the US military’s nerve agent antidote devices of the 1970s and the first commercial adrenaline (epinephrine) pens in the 1980s, is synonymous with compression spring-powered, needle-based drug delivery.
“Ideally, a platform’s functionality provides a maximum range of drug volume and viscosity capability, minimum cost, and time to customise for each application.”
Compression springs offer a simple and inexpensive energy storage option. The power density is high, meaning the energy can be released very quickly. However, the energy density is very low; in other words, the more work required, the larger the spring must be. It is also difficult to control the energy release once it is activated and some potential energy is converted to kinetic energy by the spring mass, which can pose challenges such as loud noises on activation and glass syringe breakage at the end of the motion.
The continued trend towards patient-use devices has led to a projected 18.1% compound annual growth rate to 2027 for the autoinjector market.1 As the number of drugs – especially biologics – and patient dosing regimens using autoinjectors expands, so too has the number of available options in injection platforms and power sources, all in pursuit of an improved patient experience and greater range of applications for a single platform.
One approach is to control the energy release of a compression spring, as is the case with the Controllable Force Autoinjector™ (CFAI) developed by Battelle.2 Presentations on the CFAI, as shown in Figure 1, have shown the ability to manage a 5 N needle insertion force and a controlled 22–30 N force during delivery of 1 mL at 125 cP over approximately 45 mm of plunger travel. Meanwhile, a 25 N spring in a comparable conventional autoinjector application provided approximately 20–22 N during needle insertion and declined to approximately 10 N at the end of 45mm plunger travel. The CFAI provides a much more desirable force profile during both needle insertion and drug delivery.
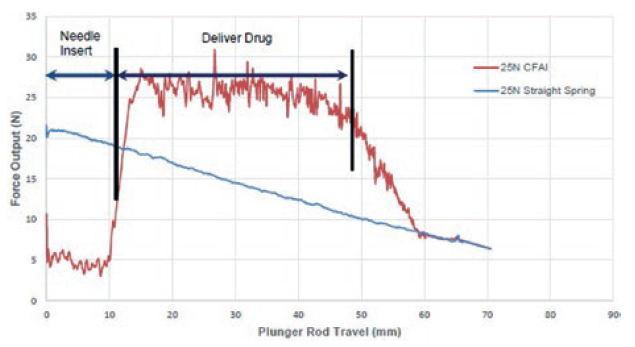
Figure 1: CFAI bench testing data, courtesy of Battelle. © 2022 Battelle Memorial Institute. All rights reserved.
Another example, breaking away from the fully disposable model, is the Phillips-Medisize reusable electronic autoinjector,3 which is capable of delivering a range of volumes and viscosities by configuring its battery-powered reusable motor drive system, which interfaces with disposable cassettes containing a 1.0 or 2.25 mL prefilled syringe. The reusable electronic drive module changes the economics case for connected autoinjectors, and the disposable cassette reduces environmental waste versus a fully disposable autoinjector.
“In addition to bowl feeding to an assembly station, the compact gas cylinder form factor also supports bulk packing to minimise shipping and storage costs, as well as laser marking to provide traceability and assembly mistake-proofing.”
Similarly, in cases where an autoinjector may not be the ideal drug delivery device and an on-body injector (OBI) may be preferred, there are emerging alternatives to springs that offer benefits in long-term delivery across a range of viscosities and volumes. These options include the Subcuject osmotic drive system,4 which has broad flexibility in drug viscosity (as discussed in the referenced article). Another concept offered by Battelle is its compact, non-linear ChemEngine™ drive system.5
In addition to spring controls and the alternative drives already discussed, great strides in compressed gas innovation have resulted in another emerging option for drug delivery device power sources. In the past, industrial gas cylinders, which at present have leak, size and activation issues, were the only option. Picocyl, a medical components company, has solved these problems, producing gas cylinders with the quality and reliability necessary for medical applications. Medical-grade compressed gases have been used in precision applications, such as cataract surgery, for several years and, more recently, these gas cylinders have also been used in autoinjectors,6 OBI transfer devices,7 specialty oral dosages8,9 and nasal delivery.
COMPRESSED GAS AND BROAD PLATFORM CAPABILITY
The primary purposes of a drug delivery platform include reuse of device design, test data, human factors and manufacturing assets across a range of drug formulations with varying properties. Commonality across platform variants and flexibility to drug-specific requirements is the balance to be achieved. A well-designed and proven platform reduces development and manufacturing costs, while providing predictable human factors and patient tolerability outcomes. These are all critical factors for reducing device risk in a drug development programme.
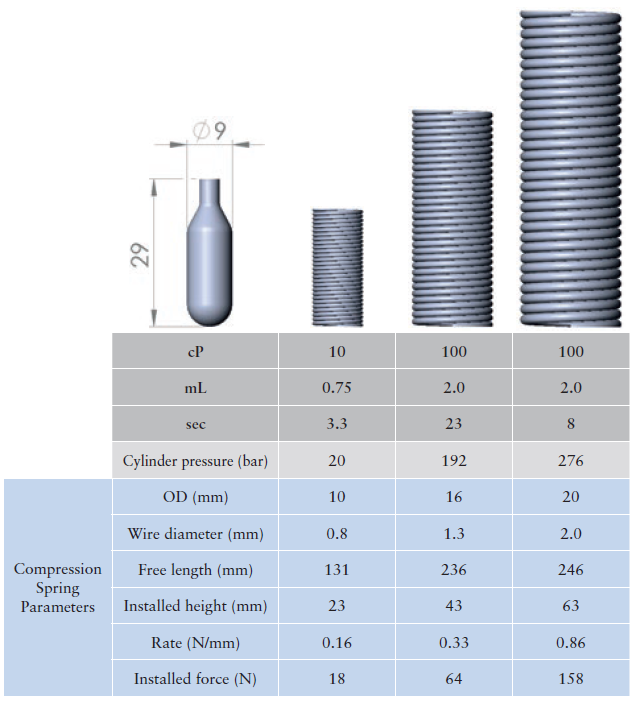
Figure 2: 1.0 mL cylinder in three volume and viscosity scenarios, using 1.0, 2.25 and 2.25 mL syringes, respectively, on a Picocyl test bench, shown with comparable compression springs.
Ideally, a platform’s functionality provides a maximum range of drug volume and viscosity capability, minimum cost, and time to customise for each application, and minimum effort to manage autoinjector variants once in commercial production.
As shown in Figure 2, a compressed gas cylinder of fixed size can be manufactured to contain different pressures, customised to suit the injection force requirements. In the visual representation, a cylinder used in an autoinjector system designed for cylinders up to 276 bar delivers the same force as springs that are physically much larger while requiring more space.
It is critical to note that, while the cylinder itself may be safely loaded to 276 bar or more, the autoinjector itself does not need to withstand the same pressure. Once pierced, the gas first expands into a designed “dead space” before applying pressure to a plunger and, therefore, the device and surrounding components are never under the same pressure at the cylinder.
Additional flexibility can be achieved by tailoring the gas type to the application. Autoinjectors, in practice, can be used across a wide range of temperatures, from refrigerated storage temperatures to high temperatures in automobiles, baggage or on-person. In this application, pure gases, such as argon or nitrogen, are appropriate as the gas pressure varies as a function of the absolute temperature. Conversely, the vapour pressure of liquified gases, such as HFC 134, vary significantly. For example, from 0–40°C, the pressure of argon will increase by 15%, while the pressure of HFC 134 will increase by 150%.
Liquified gases, however, are well suited to applications where relatively constant pressure is desired over a long stroke and the temperature does not vary widely, such as OBIs and surgical devices where the temperature is regulated by body temperature or the environmental controls of an operating room. In these applications, liquified gases, such as liquified carbon dioxide, provide higher expansion volumes at high pressures than pure gases.
High pressure gas cylinders can be filled with naturally occurring gases, such as argon and carbon dioxide, which are environmentally friendly compared with fluorinated gases.
HUMAN FACTORS
The same flexibility offered by compressed gases in generating pressure can also extend to human factors, such as noise, vibration and delivery time. Upon activation, the cylinder is opened, releasing gas into the dead space and, once sufficient pressure is achieved, starts moving the plunger. The associated noise and vibration during this process is limited to the opening of the cylinder and the movement of the plunger.
Compressed gas cylinders also offer customisation of delivery time to accommodate patient tolerability and drug absorption, adjusting to application factors such as temperature, volume, viscosity, body location and subcutaneous versus intramuscular injection. As shown in Figure 3, varying the cylinder pressure in these four volume-viscosity scenarios provides a broad range of injection times – including longer times that may be better suited to an OBI than an autoinjector – that offer customisation to patient needs.
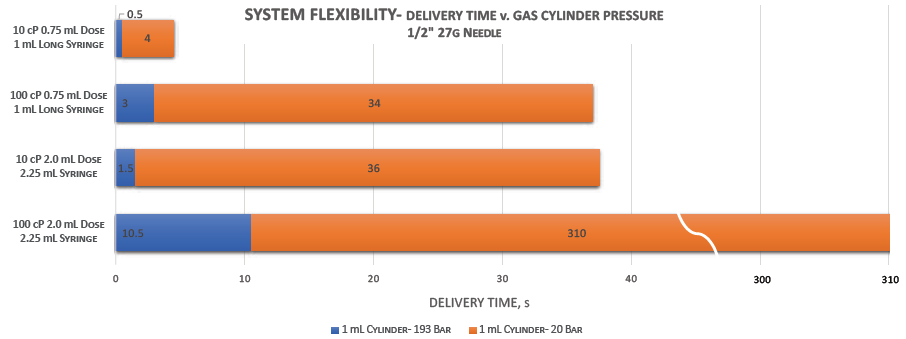
Figure 3: Potential range of delivery times for 1.0 mL cylinders with varied pressures across delivered volumes and viscosities.
INJECTION PLATFORM CONSIDERATIONS: DESIGN FOR THE MAXIMUM EXPECTED CYLINDER PRESSURE TO PROVIDE MAXIMUM PLATFORM FLEXIBILITY
As noted previously, an ideal platform provides a maximum range of drug volume and viscosity capability, minimum cost, and time to customise for each application, and a minimum effort to manage autoinjector variants once in commercial production. Building on the first need (maximum range of drug volume and viscosity), the data modelled in Table 1 projects the dose volume that can be delivered for viscosities of 10 and 100 cP, at a minimum of 2 seconds and a maximum of 20 seconds. The intention here is not to suggest the volumes suitable for a specific drug or within the patient tolerability range but to demonstrate the very broad operating window for a compressed gas-powered autoinjector.
cP | sec | mL |
10 | 2 | 3.4 |
10 | 20 | 12 |
100 | 2 | 1.3 |
100 | 20 | 3.5 |
Table 1: Range of injection volumes and viscosities in a 2–20 second time frame for a device designed for 1.0 mL 270 bar cylinder pressure. Picocyl test bench data.
The isolated pressure within the cylinder, not applied to the device until activation, also offers other benefits compared with springs, including lower stress on the assembled device, no plastic creep within the device subsystems and no energy loss due to stress relaxation in the spring resulting from long shelf-life storage under high compression.
DOWNSTREAM BENEFITS OF POWER SOURCE SIZE STANDARDISATION
The benefits of a platform with flexibility in volume and viscosity are not limited to the drug and the patient, but also the unit cost. New or modified autoinjector components must consider the following downstream impacts to cost and risk:
- Component tooling build cost and ongoing maintenance
- Component tooling validation
- Component manufacturing set-up complexity
- Component inventory management
- Assembly process equipment build cost and ongoing maintenance
- Assembly process validation
- Assembly process manufacturing set-up (i.e. changeover complexity)
- Assembly inventory management.
- The use of a platform in which a single variable (gas pressure in the selected cylinder size) provides capability across a broad range of drug volumes and viscosities provides a less complex and lower cost supply chain for both component tooling and validation and downstream assembly equipment and validation. Furthermore, there are assembly process benefits to a single cylinder size installed via a simple bowl feed to a “pick and place” robotic operation, compared with a platform with multiple spring options and the associated complexity of feeding and assembly to compress and retain the spring during subsequent assembly operations.
In addition to bowl feeding to an assembly station, the compact gas cylinder form factor also supports bulk packing to minimise shipping and storage costs, as well as laser marking to provide traceability and assembly mistake-proofing.
SUMMARY
Decades of autoinjector success have been enabled by and dependent upon compression springs. However, today, there are options to improve the spring force profile and alternatives to the use of springs altogether. Compressed gases represent the next evolution of spring alternatives, offering broad injection platform capability and flexibility, matched with minimised downstream one-time and ongoing costs of platform variants (Box 1).
BOX 1: BENEFITS OF COMPRESSED GAS-POWERED INJECTION DEVICES
- Define a broad volume-viscosity platform around a single canister size.
- Ease of gas and pressure selection in the same canister to match specific drug volumes, viscosities and human factors (i.e. platform variants).
- Low one-time development costs for platform variants.
- Laser-marked traceability, assembly error-proofing and bowl feeding enabled by canister form factor.
- Low one-time industrialisation costs for assembly of platform variants.
- Ease of production assembly changeover between platform variants.
REFERENCES
- Sonar A, Sumant O, “Allied Market Research, Autoinjectors Market by Type (Disposable Autoinjectors and Reusable Autoinjectors), Application (Rheumatoid Arthritis, Anaphylaxis, Multiple Sclerosis, and Others), and End user (Homecare Settings and Hospitals & Clinics): Global Opportunity Analysis and Industry Forecast, 2020–2027”. Allied Market Research, Sep 2020.
- Atterbury W, “Introducing Controllable Force Autoinjector (CFAI)”, Presentation at Drug Delivery West Conference (PODD), May 2018.
- Sørensen B, “Re-usable Electronic Autoinjector – Flexible Performance”, ONdrugDelivery, May 2021, Issue 120, pp 44–46.
- Schmidt Moeller C, “Subcuject WBI: Low-Cost, Larger Volume, High-Viscosity Wearable Bolus Injector – Using Standard Glass Primary Packaging”, ONdrugDelivery, May 2021, Issue 120, pp 58–60.
- Lafreniere A, “Enabling Reduced Size in On-Body Delivery”. Presentation at Partnership Opportunities in Drug Delivery (PODD), October 2020.
- Roe MJ, “Challenges in High-Viscosity, High-Volume Drug Delivery”. ONdrugDelivery, Oct 2021, Issue 125, pp 40–43.
- “New Utility Patent and enFuse® Vial Transfer System Technology”. Enable Injections. Company web page. Retrieved Apr 6, 2020.
- Progenity. (2021, October 13). “Progenity Announces Several Patents Granted by USPTO, Strengthening the Company’s Intellectual Property Position in Ingestible Therapeutics Technologies”. Press Release, Progenity, Oct 13, 2021.
- Shimizu et al, (2021). Ingestible device for delivery of therapeutic agent to the gastrointestinal tract (US Patent No. 11,007,356). US Patent and Trademark Office.